1H NMR-based assay for lysine demethylase LSD1 and its application to inhibitor screening
Original Research Paper
Hui Wang, Minghui Shu, Xiaofan Li, Wei-Guo Zhu, He Wen & Zebin Mao
Genome Instability & Disease , 2 302–308 (2021)
Abstract
Lysine-specific demethylase 1 (LSD1) with oxidation activity that relies on flavin adenine dinucleotide (FAD) to subsequently produce formaldehyde have been associated with various types of cancers. Here, we report an NMR-based assay for detecting the LSD1 enzyme activity. Our approach can be performed in a reaction buffer without any extraction step, achieve a time-dependent absolute quantification, and be useful for the enzyme inhibitor screening. This precise assay allows us to develop new inhibitors of the LSD1 as anticancer agents.
Introduction
Epigenetics is defined as heritable changes in gene expression that are independent from structural DNA sequence, but rather chemical alteration within the chromatin (Zhu & Reinberg, 2011). Chromatin is the macromolecular structure that mainly contains DNA and histone. The basic unit of chromatin is the nucleosome, which is composed of DNA wrapped around two copies of four histones (H2A, H2B, H3, and H4) (Etchegaray & Mostoslavsky, 2016). The N-terminal tails of histones can be modified by the post-translational modifications (PTMs), which influence variety nuclear processes including transcription, DNA replication, and DNA repair (Xu et al., 2016). In general, histone modifications mainly include acetylation, phosphorylation, ubiquitination, and methylation (Zhang et al., 2015). In past few decades, a number of evidences have revealed that histone lysine methylation is associated with chromatin remodeling, transcriptional regulation, X-chromosome inactivation, and genomic imprinting (Jambhekar et al., 2019; Zhang & Reinberg, 2001). Methylation of lysine residues within histone is regulated by methyltransferases (KMTs) and demethylases (KDMs). So far, more than 20 mammalian KDMs have been identified (Kooistra & Helin, 2012).
Among them, the first characterized protein of KDM is lysine-specific demethylase 1 (LSD1/KDM1/KIAA0601/BHC110/AOF2), which belongs to the flavin-containing oxidases family (Shi et al., 2004). LSD1 is able to catalyze the demethylation of mono- or di-methylated histone H3 lysine4 (H3K4me1/2) via a flavin adenine dinucleotide (FAD)-dependent oxidation (Shi, 2007). During the enzymatic reaction of LSD1, the cofactor FAD is turned over to its reduced form FADH2, which is re-oxidized by oxygen to generate hydrogen peroxide, and formaldehyde and amine are finally produced (see Fig. 1). Many studies indicated that LSD1 is overexpressed in various human cancers, including lung, breast, liver, colon, and blood cancer (Jie et al., 2013; Kim, 2021; Lv et al., 2012; Serce et al., 2012; Wouters et al., 2009). In addition, the expression levels of LSD1 are significantly enhanced in pluripotent germ cell tumor cells, such as teratocarcinoma, embryonic carcinoma, and seminoma (Wang et al., 2011). These studies suggest that LSD1 plays an important role in tumorigenesis and can be a therapeutic target for the treatment of cancer. Therefore, the inhibitors of LSD1 are necessary for dissection its functional importance in the cellular processes and development of the new anticancer drugs (Hojfeldt et al., 2013).
Fig. 1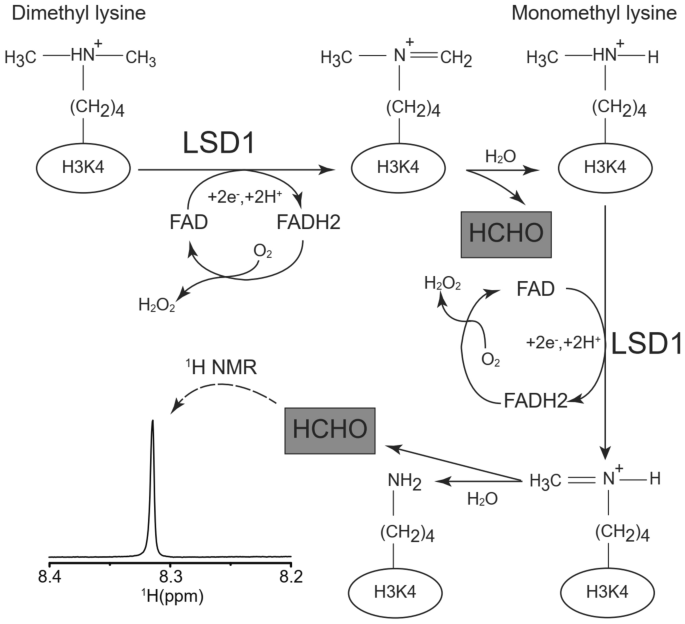
Reaction of LSD1 and 1H-NMR chemical shift for detection of LSD1 activity. The proposed mechanism for histone lysine demethylation mediated by flavin adenine dinucleotide (FAD)-dependent oxidase LSD1. The product of LSD1 enzyme reaction, formaldehyde, was indicated with gray boxes. Representative 1H-NMR spectrum of formaldehyde (0.5 mM) and its chemical shift in reaction buffer (pH = 8.5) were also presented
Full size imageTo data, various high-throughput screening (HTS) platforms have been utilized to probe for small molecule inhibitors of LSD1, including the antibody-based assays (Gauthier et al., 2012; Wang et al., 2012), the coupled enzyme-based assays (Yu et al., 2014), and the mass spectrometry-based assays (Plant et al., 2011). Given high sensitivity, the antibody-based assays are susceptible to quenching interference by compounds. Therefore, elimination of the artifacts from detected signals is necessary. Though the additional wash steps meet the requirement, however, it needs time and large amounts of peptide substrates. The mass spectrometry approach is able to directly detect the demethylated products with high sensitivity. However, it requires preparation of an optimized substrate, additional sample extraction steps to remove useless components, and several samples for investigation of time-dependent enzyme activity. In the coupled enzyme-based assay, the small byproduct formaldehyde is used to determine the enzymatic turnover of the LSD1. Formaldehyde dehydrogenase (FDH) is used to oxidize formaldehyde into formic acid, thereby reducing NAD + into NADH, which can be monitored by absorbance at 340 nm. However, it requires exclusion of the artifacts from the coupled enzyme reaction, which leads to false positives and negatives.
Therefore, a convenient and precise assay that can directly measure the product and evaluate the enzyme activity of LSD1 is needed.
Results
In the current study, we proposed a novel approach that can directly detect the product (formaldehyde) of LSD1-mediated lysine demethylation reaction. Formaldehyde contains structural aldehyde, where the proton is covalently connected with ketonic-CO, specifically appears as a singlet on range from 8 to 9 ppm in one-dimensional proton NMR spectrum. In contrast, protons on carbon (i.e. alkyl, benzylic or aromatic) or protons on oxygen/nitrogen (i.e. alcohol, phenol, or amine) generally appeared on the range from 0 to 8 ppm or 9 to 13 ppm in 1H-NMR spectrum, respectively. Though the pH degree of solution may influence the chemical shift of proton, we characterized that aldehyde proton of formaldehyde in the reaction buffer (pH = 8.5) presented on 8.32 ppm in 1H-NMR spectrum (Fig. 1). We also tried other standard compounds without aldehyde structure, such as leucine, tyrosine, and ethanol, found that no peaks were appeared on the range from 8 to 9 ppm (data not shown), indicating that our approach can be selectively detect the aldehyde proton of formaldehyde.
Due to high selective aldehyde proton of formaldehyde, we applied our approach in the reaction buffer. FAD and the purified histone fragmentation were incubated in the reaction buffer without or with recombinant wild-type (WT) LSD1 and inactive mutant LSD1 (K661A) (Stavropoulos et al., 2006) at 37 °C for 12 h, separately. As shown in the Fig. 2a, no observable signals of formaldehyde were presented in the case of the WT-LSD1 absent (top in Fig. 2a) or the mutant LSD1 (K661A, middle in Fig. 2a), respectively. In contrast, WT-LSD1 gave rise to a clear signal of formaldehyde (bottom in Fig. 2a). This result indicated that our assay is highly specific for LSD1 demethylation activity and that the detected peak is not produced by non-enzymatic conversion of substrate. After the enzyme reaction, we carried out western blot with relative antibodies to confirm the protein levels of the methylated H3K4. As shown in Fig. 2b, WT-LSD1 effectively reduced the protein levels of the di- and mono-methylated H3K4 and insignificantly changed that of the pan-acetylated H3, validating the application of our assay in the reaction buffer.
Fig. 2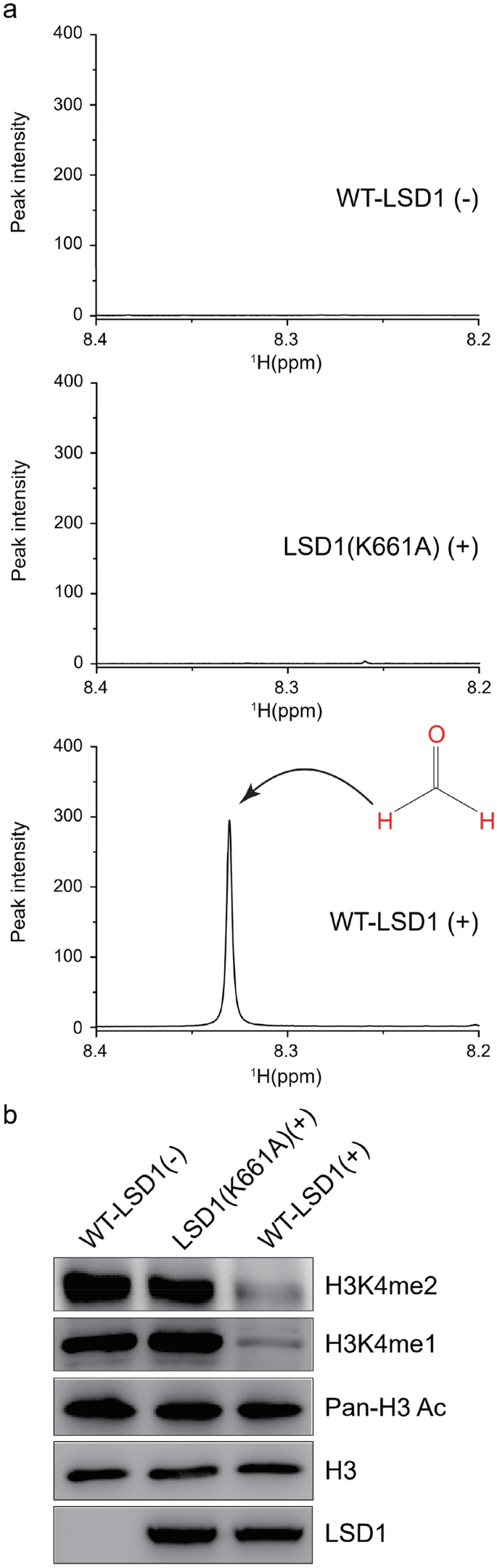
LSD1-mediated demethylation reactions. a 1H-NMR spectra of formaldehyde in the reaction buffer in the absence of WT-LSD1 (top panel) or presence of the inactive mutant LSD1 (K661A) (middle panel) and WT-LSD1 (bottom panel). Each experimental buffer contains flavin adenine dinucleotide (FAD) (20 μM), recombinant LSD1 protein (10 μg), and purified histone fragmentation (20 μg). b After reaction, the expression levels of mono- and di-methylated H3K4, histone H3, pan-acetylated H3, and LSD1 were monitored by Western blotting with specific antibodies indicated on the right. Samples were obtained from absence of WT-LSD1 or presence of the inactive mutant LSD1 (K661A) and WT-LSD1, separately
Full size imageThe assay also allowed to be applied in the real-time monitoring of the product generation due to the non-destructive nature of the NMR strategy. In contrast, other approaches require destructive sample extraction and various samples are needed for reflection of time-dependent enzyme activity. We carried out real-time monitoring of LSD1 enzyme reaction and observed time-dependent generation of product (Fig. 3a). For characterization of the enzyme kinetic reaction, the absolute quantification of product is required. Thus, we obtained several 1H-NMR spectra of formaldehyde with the known concentration and its serial diluents. The correlation between peak intensities of formaldehyde on 1H-NMR and diluted concentration was outstanding and provided calibration curve for the absolute quantification (Fig. 3b). With exact measurement of the products, we can calculated the LSD1 enzyme activity in “nmole of products/min/mL” (= milliunit/mL) units, which is generally used in the enzyme kinetics (Fig. 3c). The precise enzyme activity of LSD1 we used is 0.52 ± 0.036 milliunit/mL.
Fig. 3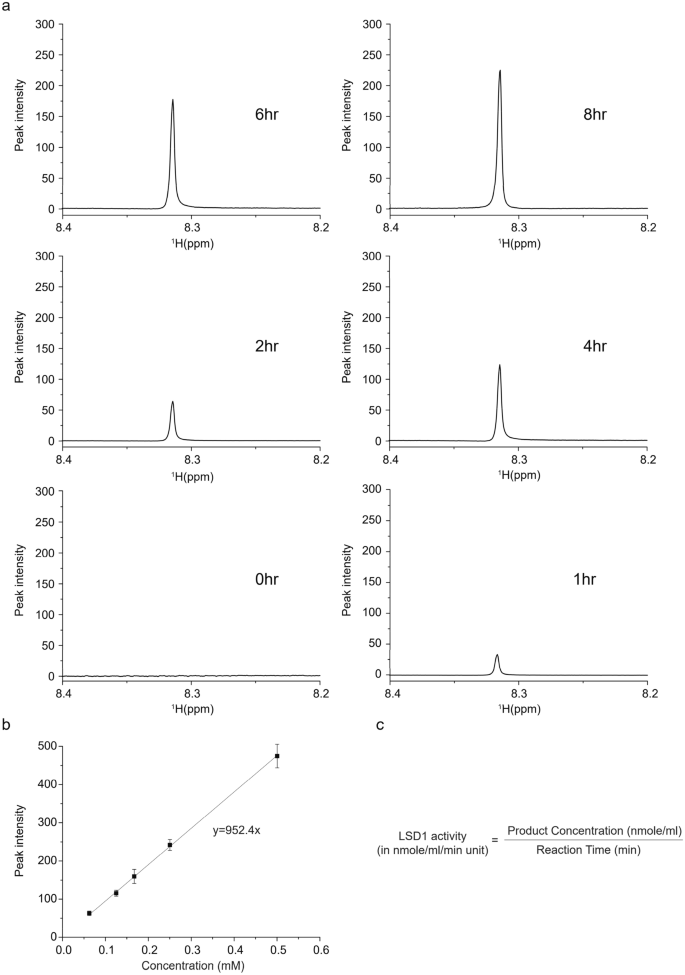
Time course changes of the product of LSD1 and the absolute quantification of formaldehyde. a Time-dependent monitoring of the generation of the product of LSD1 according to the indicated time. Three independent experiments were performed. b Correlation between 1H-NMR peak intensity and absolute concentration of formaldehyde. Three independent experiments were performed. Error bars represent standard deviation. c Equation for the enzyme activity calculation
Full size imageAn ultimate goal of the enzymatic assay is application to the inhibitor screening. Given the validation of the promising utility of our NMR-based assay, we applied it to detect inhibition effect of LSD1 activity by a known inhibitor. When we added a LSD1 inhibitor GSK2879552 (Mohammad et al., 2015) with several different concentrations, such as 2 μM (Fig. 4a), 1 μM (Fig. 4b), and 0.5 μM (Fig. 4c), the 1H-NMR signal intensity of formaldehyde was dose-dependently reduced (Fig. 4a–c), as opposed to remain unchanged peak in control group (top left in Fig. 4). Subsequently, this concept of inhibitor screening was actually tested with several cell membrane-permeable compounds from natural sources. As shown in Fig. 4d–j, the compounds were appeared different inhibitory efficacy (Fig. 4d–j). This result indicated that our NMR-based assay can be applied in inhibitor screening studies. Because our approach does not require any sample preparation steps after the enzyme reaction, the reaction can be performed in the NMR tube itself and the spectra can be obtained using an automatic sample changer. Because one NMR measurement takes only 2 min, ∼7200 compounds can be screened per day if 10 compound mixtures are screened in a single NMR tube.
Fig. 4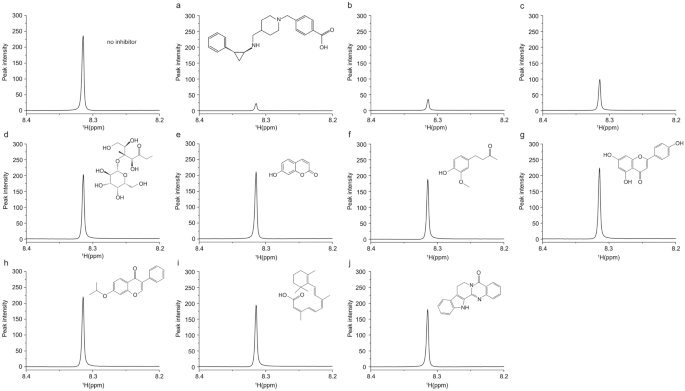
Enzyme inhibition assay. 1H-NMR spectra of product of LSD1 in the reaction buffer without inhibitor (left top) or with the known enzyme inhibitor GSK2879552 with various concentrations, such as 2 μM a, 1 μM b, 0.5 μM c and 10 μM of each natural compound named lactulose d, umbelliferone e, vanillylacetone f, apigenin g, Ipriflavone h, Isotretinoin i, and rutaecarpine j, separately. Before the enzymatic reaction, the buffer was added 10 μg of the recombinant LSD1 and the related inhibitors and natural compounds, and then the mixture was pre-incubated at 37 °C for 5 min. After incubation, 20 μM of flavin adenine dinucleotide (FAD) and 20 μg of the purified histone fragmentation were added and incubated then at 37 °C 12 h before NMR measurement. The structure of each natural compound is shown above its NMR spectrum
Full size imageOverall, our NMR-based assay is readily to perform and specific for the FAD-dependent oxidase LSD1. The NMR method can directly detect the formaldehyde without any extraction steps, resulting in shortening experimental procedure, eliminating interference of artifacts, and reducing sources of sample variations. The NMR-based approach can also be applied to detailed enzyme kinetic studies and real-time monitoring of the enzyme reaction due to its non-destructive nature. Because LSD1 is directly associated with carcinogenesis, our NMR-based assay may help to facilitate the development of new inhibitors for LSD1 as anticancer agents.
Materials and methods
Chemicals and reagents
The flavin adenine dinucleotide (FAD) and GSK2879552 were purchased from Achieve Perfection Biology (Boston, MA, USA).
Histone acid extraction
Histone was extracted as previously described (Cao et al., 2016). Briefly, HeLa cell pellets were lysed in hypotonic buffer, re-suspended in 0.4 M sulfuric acid and precipitated by trichloroacetic acid. The histone pellet was washed with acetone and dissolved in ddH2O.
Cloning of LSD1 and its mutant
The GST-LSD1 plasmid was kindly provided by Dr. Bin Peng (Shenzhen University, Guangdong Province, China). The site directed mutagenesis of the inactive mutant LSD1 (K661A) was performed using QuikChange mutagenesis kit (SBS Genetech Co) following the manufacturer's instruction. The correctness of the clones was confirmed by DNA sequencing (BGI-Shenzhen, China).
Expression and purification of LSD1 and its mutant
GST-tagged plasmids were transformed into Escherichia coli BL21 cells (TianGen, China) and induced with 0.1 mM IPTG (Sigma Aldrich, USA) overnight at 25 °C and then purified using glutathione–Sepharose 4B beads (GE Healthcare, USA). The GST -tag was cleaved with HRV 3C protease (Takara Bio, Otsu, Japan) overnight at 4 °C and applied to a size-exclusion column.
Western blotting
After enzyme reaction, the whole samples were added 5X SDS sample loading buffer and boiling at 100 °C for 5 min. Immunoblotting procedures were performed as previously described (Li et al., 2018). Histone proteins were sized fractionated on a 15% SDS-PAGE gel. The antibodies used in this study include: anti-H3K4me2 (ab11946) and anti-histone H3 (ab176842) from Abcam; Anti-H3K4me1 (A2355) from Abclonocol; anti-Histone H3ac (pan-acetyl, 39139) and anti-LSD1 (61607) from Active-Motif.
Sample preparation for NMR spectroscopy
The reactions were carried out in a buffer state (50 mM Tris–HCl pH 8.5, 50 mM KCl, 5 mM MgCl2). Before the enzymatic reactions, 100 μL of buffer was added 10 μg of the recombinant wild-type or mutant LSD1 proteins, 20 μM of flavin adenine dinucleotide (FAD), and 20 μg of purified histone fragmentation, and then adjusting with the reaction buffer to reach final volume 200 μL. For inhibitor screening assay, the recombinant LSD1 was pre-incubated with the related inhibitors at 37 °C for 5 min before the reaction. The mixtures were incubated then at 37 °C 8–12 h before NMR measurement.
NMR measurement
NMR spectra were measured on a Bruker ASCEND 600 MHz NMR magnet system (Bruker, Fallanden, Switzerland) equipped with cryogenic probes. The acquisition parameters for one-dimensional NMR spectra were essentially the same as those previously reported (Wen et al., 2015). All one dimensional NMR spectra were obtained from pre-noesysat pulse sequence (noesygppr1d) with 128 number of scan (NS). The time domain data were Fourier transformed, phase corrected, and baseline corrected manually. The time-dependent changes of one dimensional proton NMR spectra for formaldehyde were obtained at 310 K for 8 h.
Availability of data and materials
Not applicable.
Code availability
Not applicable.
References
Cao, L. L., Wei, F., Du, Y., Song, B., Wang, D., Shen, C., Lu, X., Cao, Z., Yang, Q., Gao, Y., et al. (2016). ATM-mediated KDM2A phosphorylation is required for the DNA damage repair. Oncogene, 35, 301–313. https://doi.org/10.1038/onc.2015.81
Etchegaray, J. P., & Mostoslavsky, R. (2016). Interplay between metabolism and epigenetics: A nuclear adaptation to environmental changes. Molecular Cell, 62, 695–711. https://doi.org/10.1016/j.molcel.2016.05.029
Gauthier, N., Caron, M., Pedro, L., Arcand, M., Blouin, J., Labonte, A., Normand, C., Paquet, V., Rodenbrock, A., Roy, M., et al. (2012). Development of homogeneous nonradioactive methyltransferase and demethylase assays targeting histone H3 lysine 4. Journal of Biomolecular Screening, 17, 49–58. https://doi.org/10.1177/1087057111416659
Hojfeldt, J. W., Agger, K., & Helin, K. (2013). Histone lysine demethylases as targets for anticancer therapy. Nature Reviews Drug Discovery, 12, 917–930. https://doi.org/10.1038/nrd4154
Jambhekar, A., Dhall, A., & Shi, Y. (2019). Roles and regulation of histone methylation in animal development. Nature Reviews Molecular Cell Biology, 20, 625–641. https://doi.org/10.1038/s41580-019-0151-1
Jie, D., Zhongmin, Z., Guoqing, L., Sheng, L., Yi, Z., Jing, W., & Liang, Z. (2013). Positive expression of LSD1 and negative expression of E-cadherin correlate with metastasis and poor prognosis of colon cancer. Digestive Diseases and Sciences, 58, 1581–1589. https://doi.org/10.1007/s10620-012-2552-2
Kim, T. (2021). LncRNAs as key players in the MYC pathways. Genome Instability & Disease, 2, 24–38. https://doi.org/10.1007/s42764-021-00032-3
Kooistra, S. M., & Helin, K. (2012). Molecular mechanisms and potential functions of histone demethylases. Nature Reviews Molecular Cell Biology, 13, 297–311. https://doi.org/10.1038/nrm3327
Li, Y., Li, Z., Dong, L., Tang, M., Zhang, P., Zhang, C., Cao, Z., Zhu, Q., Chen, Y., Wang, H., et al. (2018). Histone H1 acetylation at lysine 85 regulates chromatin condensation and genome stability upon DNA damage. Nucleic Acids Research, 46, 7716–7730. https://doi.org/10.1093/nar/gky568
Lv, T., Yuan, D., Miao, X., Lv, Y., Zhan, P., Shen, X., & Song, Y. (2012). Over-expression of LSD1 promotes proliferation, migration and invasion in non-small cell lung cancer. PloS One, 7, e35065. https://doi.org/10.1371/journal.pone.0035065
Mohammad, H. P., Smitheman, K. N., Kamat, C. D., Soong, D., Federowicz, K. E., Van Aller, G. S., Schneck, J. L., Carson, J. D., Liu, Y., Butticello, M., et al. (2015). A DNA hypomethylation signature predicts antitumor activity of LSD1 inhibitors in SCLC. Cancer Cell, 28, 57–69. https://doi.org/10.1016/j.ccell.2015.06.002
Plant, M., Dineen, T., Cheng, A., Long, A. M., Chen, H., & Morgenstern, K. A. (2011). Screening for lysine-specific demethylase-1 inhibitors using a label-free high-throughput mass spectrometry assay. Analytical Biochemistry, 419, 217–227. https://doi.org/10.1016/j.ab.2011.07.002
Serce, N., Gnatzy, A., Steiner, S., Lorenzen, H., Kirfel, J., & Buettner, R. (2012). Elevated expression of LSD1 (Lysine-specific demethylase 1) during tumour progression from pre-invasive to invasive ductal carcinoma of the breast. BMC Clinical Pathology, 12, 13. https://doi.org/10.1186/1472-6890-12-13
Shi, Y. (2007). Histone lysine demethylases: Emerging roles in development, physiology and disease. Nature Reviews Genetics, 8, 829–833. https://doi.org/10.1038/nrg2218
Shi, Y., Lan, F., Matson, C., Mulligan, P., Whetstine, J. R., Cole, P. A., Casero, R. A., & Shi, Y. (2004). Histone demethylation mediated by the nuclear amine oxidase homolog LSD1. Cell, 119, 941–953. https://doi.org/10.1016/j.cell.2004.12.012
Stavropoulos, P., Blobel, G., & Hoelz, A. (2006). Crystal structure and mechanism of human lysine-specific demethylase-1. Nature Structural & Molecular Biology, 13, 626–632. https://doi.org/10.1038/nsmb1113
Wang, C., Caron, M., Burdick, D., Kang, Z., Auld, D., Hill, W. A., Padros, J., & Zhang, J. H. (2012). A sensitive, homogeneous, and high-throughput assay for lysine-specific histone demethylases at the H3K4 site. Assay and Drug Development Technologies, 10, 179–186. https://doi.org/10.1089/adt.2011.0395
Wang, J., Lu, F., Ren, Q., Sun, H., Xu, Z., Lan, R., Liu, Y., Ward, D., Quan, J., Ye, T., et al. (2011). Novel histone demethylase LSD1 inhibitors selectively target cancer cells with pluripotent stem cell properties. Cancer Research, 71, 7238–7249. https://doi.org/10.1158/0008-5472.CAN-11-0896
Wen, H., Xu, W. J., Jin, X., Oh, S., Phan, C. H., Song, J., Lee, S. K., & Park, S. (2015). The roles of IP3 receptor in energy metabolic pathways and reactive oxygen species homeostasis revealed by metabolomic and biochemical studies. Biochimica et Biophysica Acta, 1853, 2937–2944. https://doi.org/10.1016/j.bbamcr.2015.07.020
Wouters, B. J., Lowenberg, B., Erpelinck-Verschueren, C. A., van Putten, W. L., Valk, P. J., & Delwel, R. (2009). Double CEBPA mutations, but not single CEBPA mutations, define a subgroup of acute myeloid leukemia with a distinctive gene expression profile that is uniquely associated with a favorable outcome. Blood, 113, 3088–3091. https://doi.org/10.1182/blood-2008-09-179895
Xu, W., Wang, F., Yu, Z., & Xin, F. (2016). Epigenetics and cellular metabolism. Genetics & Epigenetics, 8, 43–51. https://doi.org/10.4137/GEG.S32160
Yu, W., Eram, M. S., Hajian, T., Szykowska, A., Burgess-Brown, N., Vedadi, M., & Brown, P. J. (2014). A scintillation proximity assay for histone demethylases. Analytical Biochemistry, 463, 54–60. https://doi.org/10.1016/j.ab.2014.06.023
Zhang, T., Cooper, S., & Brockdorff, N. (2015). The interplay of histone modifications—Writers that read. EMBO Reports, 16, 1467–1481. https://doi.org/10.15252/embr.201540945
Zhang, Y., & Reinberg, D. (2001). Transcription regulation by histone methylation: Interplay between different covalent modifications of the core histone tails. Genes & Development, 15, 2343–2360. https://doi.org/10.1101/gad.927301
Zhu, B., & Reinberg, D. (2011). Epigenetic inheritance: Uncontested? Cell Research, 21, 435–441. https://doi.org/10.1038/cr.2011.26
Funding
This study was supported by National Key R&D Program of China (2017YFA0503900), National Natural Science Foundation of China (31701099, 81530074, 32090030 and 81720108027), Science and Technology Program of Guangdong Province in China (2017B030301016), the Shenzhen Municipal Commission of Science and Technology Innovation (JCYJ20200109114214463 and JCYJ20170818092450901), Shenzhen Peacock Plan, Discipline Construction Funding of Shenzhen ((2016)1452), Shenzhen Bay Laboratory (SZBL2019062801011), Science and Technology Foundation of Shenzhen City (JCYJ20170302144650949 and JCY20170818094707964), Natural Science Foundation of Guangdong Province (2017A030310459), and Natural Science Foundation of SZU (2017085).
Author information
Affiliations
Key Laboratory of Carcinogenesis and Translational Research (Ministry of Education), Beijing Key Laboratory of Protein Posttranslational Modifications and Cell Function, Department of Biochemistry and Molecular Biology, School of Basic Medical Sciences, Peking University Health Science Center, Beijing, 100191, China
Hui Wang, Wei-Guo Zhu & Zebin Mao
Guangdong Key Laboratory for Genome Stability and Human Disease Prevention, Department of Biochemistry and Molecular Biology, School of Medicine, Shenzhen University, Shenzhen, 518060, China
Minghui Shu, Wei-Guo Zhu & He Wen
College of Life Sciences and Oceanography, Shenzhen Key Laboratory of Marine Bioresource and Eco-Environmental Science, Shenzhen Key Laboratory of Microbial Genetic Engineering, Shenzhen University, Shenzhen, 518060, China
Xiaofan Li
Contributions
H.W. and H.W. designed the study and performed the experimental work. H.W., Z.M., and W.G.Z. wrote and modified the manuscript. X.L. carried out the NMR measurement. M.S. assisted in the experimental process. All authors read and approved the final manuscript.
Corresponding authors
Correspondence to He Wen or Zebin Mao.
Ethics declarations
Conflict of interest
The authors declare no potential conflicts of interest. Wei-Guo Zhu is Editor-in-Chief of Genome Instability & Disease.
Ethics approval
Not applicable.
Consent to participate
Not applicable.
Consent for publication
Not applicable.
Rights and permissions
About this article
Cite this article
Wang, H., Shu, M., Li, X. et al. 1H NMR-based assay for lysine demethylase LSD1 and its application to inhibitor screening. GENOME INSTAB. DIS. 2, 302–308 (2021). https://doi.org/10.1007/s42764-021-00051-0
Received26 July 2021
Revised18 September 2021
Accepted27 September 2021
Published09 October 2021
Issue DateOctober 2021
Share this article
Anyone you share the following link with will be able to read this content:
Get shareable linkKeywords
LSD1
NMR
Assay
Cancer
Inhibitor
用户登录
还没有账号?
立即注册