The effects of hypoxia on female reproductive system diseases
Review Article
Chenglong Ma, Zhaodian Zheng, Yanqiu Shen, Jing Fang, Xinying Huang, Jiarong Guo & Huidong Zhang
Genome Instability & Disease (2022)
Abstract
Diseases of female reproductive system include tumor diseases (e.g. breast cancer, ovarian cancer, cervical cancer, endometrial cancer, and uterine fibroids) and non-tumor diseases (e.g. endometriosis, hypertensive disorders complicating pregnancy, and fetal growth restriction). Hypoxia is one of the most important factors that may regulate the occurrence of these diseases. As a key transcription factor under hypoxia, HIF-1 (hypoxia-inducible factor-1) promotes the transcription of a vast amount of downstream genes and regulates the functions and phenotypes of various cells under hypoxia. In this review, we summarize that hypoxia and HIF-1 affect these tumor and non-tumor diseases by regulating various cell invasion, proliferation, apoptosis, autophagy, proliferation or differentiation. We also review the roles of non-coding RNAs in the regulation of various cell dysfunctions under hypoxia. We hope to reveal the roles and regulatory mechanisms of non-coding RNAs in the effects of hypoxia on female reproductive system diseases and to provide potential new approaches for early detection and clinical treatment against female reproductive system diseases.
Introduction
Due to female's physiological characteristics and their particularity of family and social responsibilities, females are facing more reproductive problems than males. Female reproductive health is not only related to women themselves, but also related to population control, population quality and social and economic development. Therefore, the protection of female reproductive health is of great significance in the realization of total human health.
Female reproductive system diseases critically threaten female reproductive health. Female reproductive system diseases include tumor-related pregnancy diseases, such as breast cancer, ovarian cancer, cervical cancer, endometrial cancer, uterine fibroids, and also include non-tumor pregnancy diseases, such as PE (preeclampsia), FGR (fetal growth restriction), endometriosis, hypertensive disorders complicating pregnancy. These female reproductive system diseases cause serious harm to women and society. However, the etiology and pathogenesis of these diseases are still largely unexplored. Increasing studies have shown that environmental factors inevitably and significantly regulate the occurrence of adverse pregnancy outcome (Meeker et al., 2009; Shields et al., 2002).
Hypoxia
Many environmental factors may induce female reproductive system diseases. Among them, hypoxia is a very important factor. Hypoxia refers to the lack of oxygen in cells and tissues due to the decrease of oxygen supply, insufficient oxygen transport or the inability of tissues to use oxygen effectively, which can lead to a variety of female reproductive system diseases. Hypoxia is very likely to occur in high-altitude areas, deep oceans, places with excessive population concentration, as well as underground tunnels built by humans. It can also occur when inhaling polluting gas mixtures (Li et al., 2021). For example, in breast tumor tissues, the mean of PO2 (oxygen partial pressure) is 2.5–28 mmHg, with a median of 10 mmHg; while the median PO2 in normal breast tissues is 65 mmHg (Vaupel et al., 2007). Over half of the breast cancer patients have PO2 lower than 2.5 mmHg (Hohenberger et al., 1998; Vaupel et al., 2007), and the lower PO2 in tumors is associated with the increased breast cancer cell metastasis and the increased mortality. In mice experiment under hypoxia, sublethal hypoxia on day 12.5 can lead to growth restriction and fetal weight loss by 26% (Ream et al., 2008). Three groups of pregnant Wistar rats received different degrees of hypoxia: 11.6%, 10.7% and 9.0%. The weight of rat embryo is significantly decreased with the decrease of oxygen supply (Grauw et al., 1986). Therefore, hypoxia plays an important role in regulation of the progression of female reproductive system diseases.
Pathophysiological mechanism of hypoxia
Several factors may lead to cell or tissue hypoxia:
① Oxygen molecules combine with hemoglobin and are transported to tissues and cells all over the body. However, CO2 molecule or other toxic molecules (e.g. CO or HCN) combine with iron ions in human hemoglobin by competing with oxygen molecules. If hemoglobin loses its function to combine with oxygen molecules, hypoxia occurs (Kunz & Ibrahim, 2003).
② In tumors, the volume of tumor increases rapidly; while the growth of blood vessels in tumor tissue is relatively slow, leading to the lack of oxygen and nutrition supply in tumor tissue (Harris, 2002). In addition, the rapid growth of tumor cells may also lead to the compression or inhibition of the growth of blood vessels, resulting in the cessation of oxygen supply by blood vessels.
③ In animal model of hypoxia, the hypoxia model can be constructed using vascular clamp. On the 14th day of rat pregnancy, the blood flow of aorta and uterus could be reduced by 40% using uterine vascular clamp. Hypertension and renal pathological changes occur in the rat mother, the invasion of trophoblast in uterus becomes insufficient, the recasting of spiral artery fails, and the placenta shows hypoxia (George et al., 2014).
④ At high altitude, the decreased oxygen concentration affects placental development of pregnant women compared with those at sea level. In the area with an altitude of 2,500 m or above, the placental morphology of pregnant women is obviously affected (Zamudio, 2003). Hypoxia environment can cause the changes of maternal adaption, and the failure of this adaptation leads to the damage of placental structure and functions (Petousi & Robbins, 1985).
Hypoxia inducible factor
HIF-1 (hypoxia inducible factor-1) is the major protein that is highly expressed under hypoxia (Fig. 1). Subsequently, HIF-1 regulates the transcription of a large number of genes for response of cells to hypoxia. HIF-1 is a heterodimer, containing an unstable ɑ subunit and a stable β subunit (Kaelin & Ratcliffe, 2008). HIF-1ɑ is unstable under normoxia but is induced by hypoxia and stably present under hypoxia. HIF-1 can bind to the promoter region of its target genes (i.e. hypoxia response element, HREs) to induce their transcription. These genes are involved in angiogenesis, glucose utilization, antioxidant stress, cell proliferation, anti-apoptosis, invasion, metastasis, etc.
Fig. 1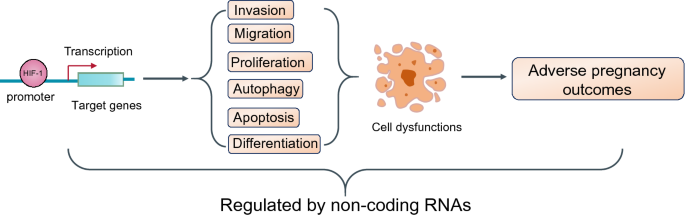
Hypoxia and HIF-1 regulate the progression of female reproductive system diseases by altering cell functions through various approaches. HIF-1, acted as a transcription factor, activates the transcription of a vast of its downstream genes, which regulate invasion, migration, proliferation, autophagy, apoptosis, and differentiation. These alterations may result in cell dysfunctions and finally induce the occurrence of female reproductive system diseases. These processes are also regulated by non-coding RNAs
Full size imageFemale reproductive system diseases include tumor-related pregnancy diseases and non-tumor pregnancy diseases. Below, we will review the effect of hypoxia, as well as HIF-1, on the progression of tumor-related female reproductive system diseases and non-tumor female reproductive system diseases. The roles of non-coding RNAs in the regulatory mechanism were also reviewed.
Effects of hypoxia on tumor-related female reproductive system diseases
Here we review the effect of hypoxia and its HIF-1 on the progression of various tumor-related pregnancy diseases. Hypoxia is closely associated with breast caner (Tang et al., 2021), ovarian cancer (Selvendiran et al., 2009), cervical cancer (Wang et al., 2018a), endometrial cancer (Chen et al., 2020a) and uterine fibroids (Ishikawa et al., 2019). The high expression of HIF-1 is significantly correlated with the short-term mortality and long-term survival rate of female tumor patients (Schindl et al., 2002). Hypoxia can regulate various functions of tumor cells, including migration, apoptosis, drug resistance, metabolism, and angiogenesis (Bensaad & Harris, 2014) (Tables 1 and 2). For example, hypoxia regulates breast cancer by altering cell invasion, proliferation and autophagy and regulates ovarian cancer by altering cell proliferation. Moreover, HIF-1 could also directly regulate cancer cell functions. In mechanisms, various non-coding RNAs play an important role in the regulations of hypoxia-induced dysfunctions of cancer cells (Fig. 2). Here, we summarize several important approaches by which hypoxia regulates dysfunctions and progression of various tumor-related pregnancy diseases.
Table 1 Hypoxia-regulated trophoblast dysfunctions and their related female reproductive system diseases
Table 2 MiRNA-regulated trophoblast dysfunctions and their related female reproductive system diseases
Full size tableFig. 2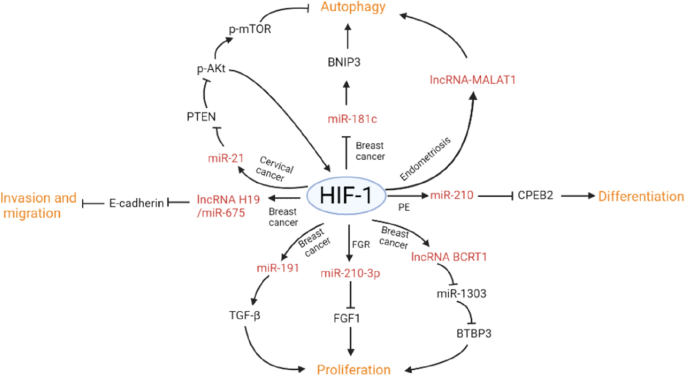
HIF-1 regulates cell functions through non-coding RNAs in various female reproductive system diseases
Full size imageHypoxia regulates cell invasion and migration
Hypoxia regulates invasion and migration in various tumor cells. In HeLa/SiHa cervical cancer cell lines, the activation of Rab11 (an important vesicle transport molecule) can promote cell invasion and migration under hypoxia, which is dependent on integrin αvβ3-mediated phosphorylation of FAK and PI3K (Xu et al., 2017). HIF-1, an important hypoxia inducible protein, can directly regulate cancer cell invasion and migration. A study on cervical cancer has shown that PLOD2 (procollagen lysine, 2-oxoglutarate, 5-dioxygenase) is induced to be highly expressed under hypoxia. In hypoxic environment, PLOD2 promotes HIF-1-mediated invasion and migration of HeLa and SiHa cells (Hofbauer et al., 2003). Under hypoxia, HIF-1 induces EMT (epithelial mesenchymal transition) by regulating the transcription of E-cadherin, SNAIL (snail family transcriptional repressor), ZEB1 (zinc finger E-box-binding homeobox 1), TWIST (twist family bHLH transcription factor) and TCF3 (transcription factor 3) (Krishnamachary et al., 2006; Moreno-Bueno et al., 2008). Recently, it has been found that hypoxia inducible proteins also include Jagged2, COX-2 (cyclooxygenase-2) and uPAR (urokinase receptor) (Jo et al., 2009; Xing et al., 2011). The changes in these molecules were found to stimulate the invasion of EMT and breast cancer cells. HIF-1 may also promote the invasion of breast cancer cells by suppressing the calcium signaling in hypoxic breast cancer cells (Davis et al., 2014). In addition, human ovarian cancer cells can up-regulate the expression of HIF-1 in hypoxic microenvironment. HIF-1 up-regulates SNAIL (snail family transcriptional repressor), inhibits the expression of E-cadherin, and promotes the invasion and migration of ovarian cancer cells (Zhang et al., 2015). Moreover, HIF-1 can also regulate the expression and accumulation of VEGF (vascular endothelial growth factor) in the nucleus through ET-1/ETAR (endothelin-1/endothelin A receptor) axis, and thus promotes the invasion of cancer cells (Cianfrocca et al., 2016). Furthermore, HIF-1 can regulate cancer cell invasion and migration through non-coding RNAs. For example, HIF-1 inhibits the expression of E-cadherin protein by up-regulating lncRNA H19/miR-675 axis, and subsequently promotes the invasion and migration of breast cancer cells (Matouk et al., 2014).
Hypoxia regulates cell proliferation
Hypoxia can regulate cancer cell proliferation. TAM (tumor-associated macrophages) are mainly located in hypoxic microenvironment of breast cancer, which is related to the poor prognosis. Mechanistically, hypoxia can increase the synthesis and secretion of galectin-3 by TAM and promote the growth and metastasis of breast cancer cells (Wang et al., 2020a). Under hypoxia, the up-regulation of MMP14 (matrix metalloproteinase 14) promotes the proliferation of TIC (tumor initiating cells) in breast cancer and further produces tumor (Hillebrand et al., 2019). The occurrence of cervical cancer is related to the up-regulation of CCL17 (chemokine ligand 17) under hypoxia. It was found that the secretion of CCL17 in HeLa and SiHa cells is increased in a time-dependent manner, and CCL17 receptor (CCR4) is also highly expressed in cervical cancer. In cervical cancer, high expression of CCL17 promotes the proliferation of cervical cancer cells through JNK (Janus kinase) and STAT5 (signal transducer and activator of transcription 5) signaling pathways (Liu et al., 2015). Other study has shown that hypoxia can synergistically interact with PTGER4 (prostaglandin E receptor 4) signaling pathway to promote the proliferation of endometrial adenocarcinoma cells and tumor growth. Compared with normal endometrium, the expression of PTGER4 in endometrial carcinoma is significantly up-regulated (Catalano et al., 2011). Moreover, hypoxia also promotes the secretion of fibroid ET-1, a powerful vasoconstrictor, in patients with uterine fibroids. ET-1 is thought to participate in the development of uterine leiomyoma by activating ETAR (endothelin A receptor) and chronic hypoxia (Tanfin & Breuiller-Fouche, 2012; Tanfin et al., 2011; Wallace et al., 2014). By interacting with ETAR, ET-1 plays an important role in tumorigenesis, such as cell proliferation, migration, invasion, transformation and angiogenesis (Wallace et al., 2018).
HIF-1 can directly regulate cancer cell proliferation. DDX3 (DEAD-box helicase 3 X-linked) is an RNA helicase that could promote cell proliferation. Hypoxia can increase DDX3 expression level. In invasive breast cancer cells, DDX3 is the direct downstream target of HIF-1 (Bol et al., 2013). In another study, bioinformatic analysis discovers that the key genes (such as TGFA, EGFR, ErbB2 and MYC) and key pathways (such as ErbB signaling pathway) are closely related to ovarian cancer cell proliferation under hypoxia. Hypoxia mainly promotes the invasion and adhesion of ovarian cancer cells by regulating HIF-1/TGFA/EGFR/ErbB2/MYC axis and also improves their proliferation ability (Zhang et al., 2018). In addition, HIF-1 can regulate the expression and accumulation of VEGF (vascular endothelial growth factor) in the nucleus through ET-1/ETAR (endothelin-1/endothelin A receptor) axis, and thus promotes the proliferation of cancer cells (Cianfrocca et al., 2016). HIF-1 can also promote cell proliferation, inhibit cell apoptosis and increase invasion by up-regulating Rab25 expression (Cheng et al., 2004). In endometrial cancer, m6A methylation-mediated HIF-1/ALKBH5/SOX2 axis can promote the expansion of endometrial cancer stem cells under hypoxia. Hypoxia activates HIF-1, which subsequently blocks the secretion of VEGF and promotes the proliferation of embryonic stem cells (Chen et al., 2014). Under hypoxia, the up-regulation of HIF-1 leads to the abnormal expression of tumor stem cell genes (such as SOX2) (Chen et al., 2020a). HIF-1 is also involved in the progression of endometrial cancer by regulating p27Kip, an inhibitor of cell cycle (Meroni et al., 2019). In uterine fibroids, antioxidant enzyme system is impaired and the expression and activity of antioxidants (e.g. CAT and SOD) are decreased. Hypoxia regulates the balance of antioxidant enzymes in myoma and myometrial cells (Fletcher et al., 2013). Hypoxia stimulates mitochondria to increase ROS (reactive oxygen species) level, and then activates the HIF-1-mediated signaling pathways, and further promotes the growth of tumor cells (Fruehauf & Meyskens, 2007).
Hypoxia can also regulate the proliferation of tumor cells through non-coding RNAs. C-Met is a receptor tyrosine kinase, which is closely related to the occurrence of ovarian cancer. Studies have shown that hypoxia can down-regulate the expression of miR-199a-3p in ovarian cancer tissues, resulting in the up-regulation of c-Met (Kinose et al., 2015). LncRNA NORAD is highly expressed in ovarian cancer tissues. Knockdown of lncRNA NORAD down-regulates the expression level of miR-199a-3p, thereby inhibiting the proliferation, invasion and migration of ovarian cancer cells (Xu et al., 2020). PTBP3 (polypyrimidine binding protein 3) is a RNA binding protein that acts as a tumor promoter in breast cancer. Hypoxia can up-regulate lncRNA BCRT1, which subsequently increases the expression of PTBP3 by inhibiting miR-1303. The up-regulated PTBP3 promotes the proliferation, migration and invasion of breast cancer cells (Liang et al., 2020). TNBC (three negative breast cancer) is a relatively high mortality subtype with high expression levels of lncRNA GHET1. Hypoxia can induce the expression of lncRNA GHET1 and activate Hippo/Yap signaling pathway, thus promoting the progression of TNBC. Knockdown of lncRNA GHET1 significantly reduces the proliferation, viability and invasion of TNBC cells, and reduces glucose consumption and lactate production (Wang & Liu, 2021). Under hypoxia, in breast cancer tissues and cells, circRNA_0001982 and MUC19 are up-regulated, while miR-1287-5p is down-regulated. Circ_0001982 enhances the expression of MUC19 in breast cancer cells through miR-1287-5p/MUC19 axis, thereby promoting glycolysis, proliferation, migration and invasion of breast cancer cells (Qiu et al., 2021).
HIF-1 can also regulates the proliferation of tumor cells through non-coding RNAs. HIF-1 and TGF-β (transforming growth factor β) signal pathway is related to cell proliferation. In breast cancer cells, hypoxia may increase the expression level of miR-191, induces miR-191 to bind with HuR (human antigen R) and leads to its down-regulation, up-regulates HIF/miR-191/TGF-β pathway, and then induces the proliferation of breast cancer cells (Nagpal et al., 2015). Other studies have reported that circRNA RNF20/miR-487a/HIF-1/HK2 (hexokinase II) axis can promote the glycolysis of breast cancer cells and promote their proliferation. In mechanism, under hypoxia, circRNF20 down-regulates miR-487a, promotes HIF-1 to bind with HK2 promoter and promotes its transcription, and ultimately promotes the development of breast cancer (Cao et al., 2020). In HeLa cells of cervical cancer, HIF-1 expression is increased under hypoxia, which up-regulates miR-152 and down-regulates the expression of WNT1 and ERBB3 in HeLa cells, and reduces the proliferation of HeLa cells (Tang et al., 2016).
Hypoxia regulates cell autophagy
Autophagy can remove the damaged cancer cells and provide nutritional sources for cell proliferation. Hypoxia promotes the development of tumor cells through autophagy. Studies have shown that hypoxia significantly increased Parkin, an E3 ubiquitin ligase, which in turn initiated mitochondrial autophagy, leading to radiation resistance in breast cancer cells. (Zheng et al., 2015). After exposure to paclitaxel, breast cancer cells activate ATF4 (activating transcription factor 4)-dependent autophagy and produce drug resistance in cancer therapy (Notte et al., 2015). On the other hand, if environment is constantly changed and is beyond the response capability of cancer cells, the level of autophagy will be unlimitedly up-regulated, eventually leading to autophagic death of cancer cells. For example, hypoxia induces autophagy in breast cancer cells. Under IR (ionizing radiation) conditions, hypoxia can improve IR-induced autophagy by inhibiting Akt/mTOR /P70S6K pathway, leading to certain radioresistance (Zhong et al., 2015). In cervical carcinoma, the signaling pathways of IRE1 (inositol required protein 1) and PERK (protein kinase RNA like Er kinase) are activated, and the expression of autophagy-related proteins are up-regulated, thus promoting cell protective autophagy (Taguchi et al., 2017).
Hypoxia can regulate cancer cell autophagy through non-coding RNAs. MiR-181c acts as a tumor suppressor to activate autophagy. Hypoxia activates BNIP3 (Bcl-2 interacting protein 3)-mediated autophagy by inhibiting miR-181c expression and improving the survival of breast cancer cells (Lee et al., 2019). HIF-1 can also directly regulate cancer cell autophagy through non-coding RNAs. The expression of miR-21 is up-regulated through PTEN/Akt/HIF-1 feedback loop. MiR-21 reduces radiation-induced autophagy of cervical cancer cells through Akt-mTOR (the mammalian target of rapamycin) signaling pathway, thus enhancing the anti-radiation ability of cervical cancer cells (Song et al., 2016).
Hypoxia regulates cancer cell differentiation
HIF-1 can regulate cancer cell differentiation. It has been found that HIF-1 is overexpressed in endometrial carcinoma, and its expression level is positively correlated with the degree of differentiation of endometrial carcinoma (Sivridis et al., 2002), suggesting that the overexpressed HIF-1 may participate in the occurrence of endometrial carcinoma and promote its development. Moreover, HIF-1 expression level is increased with the increase of the malignant degree of endometrial carcinoma.
Hypoxia regulates the progression of non-tumor female reproductive system diseases
In addition to tumor diseases, hypoxia also affects non-tumor female reproductive system diseases, including endometriosis, hypertensive disorders complicating pregnancy, fetal growth restriction, and so on (Tables 1 and 2). Hypoxia can affect the development of endometriosis by regulating cell proliferation and autophagy, affect the progression of PE by regulating cell proliferation, invasion, apoptosis and differentiation, and also affect the development of FGR by regulating cell proliferation. Moreover, HIF-1 could directly regulate cell functions under hypoxia. Furthermore, non-coding RNAs also regulate hypoxia-induced cell dysfunctions (Fig. 2). Here, we summarize several important approaches by which hypoxia regulates dysfunctions and progression of various non-tumor pregnancy diseases.
Hypoxia regulates cell invasion and migration
HIF-1 can directly regulate cell invasion and migration. The placenta of PE has a hypoxic microenvironment. Studies have shown that the level of HIF-1 is reduced after delivery of the placenta, suggesting that HIF-1 might be a predictive biomarker of PE (Akhilesh et al., 2013). HIF-1 is highly expressed in PE placental trophoblast (Rath et al., 2016), which promotes the invasion and migration of trophoblast cells. VEGF binds to the receptor on the primary cilium and activates the downstream ERK (extracellular signal-regulated kinase) signaling pathway to ensure the normal development of placenta. Under hypoxia, HIF-1 can induce the deacetylation of cilia, lead to the dissolution of cilia, destroy the signal transduction of EG-VEGF (endocrine gland-derived vascular endothelial growth factor), and then inhibit the invasion of trophoblast cells (Chen et al., 2020b). Therefore, cilia defect caused by hypoxia is one of the important factors that lead to the occurrence of PE.
Hypoxia regulates cell proliferation
Hypoxia can regulate cell proliferation. Hypoxia inhibits the expression of DUSP2 (dual-specificity phosphatase-2) and promotes the signal transduction of IL-6 (interleukin-6) and STAT3 (signal transducer and activator of transcription 3) in endometriosis cells. Subsequently, the abnormal activation of STAT3 signaling pathway promotes the proliferation of endometriosis cells (Hsiao et al., 2017). HIF-1 can also directly regulate cell proliferation. Under hypoxia, the increased level of HIF-1 up-regulates leptin expression in endometriosis tissues, promotes cell proliferation, and thus promotes the development of endometriosis (Wu et al., 2007).
Hypoxia can also regulate cell proliferation through non-coding RNAs. Some studies have shown that miR-20a is overexpressed in ESC (endometrial stromal cells) under hypoxia. The increased miR-20a leads to the decrease in DUSP2 expression level, which induces the abnormal activation of ERK and the reactivation of osteopontin and CYR61 (cysteine rich angiogenic inducer 61), which promote angiogenesis and the survival of endometriosis cells (Lin et al., 2012). Under hypoxia, miR-362-3p in HTR-8/SVneo cells is up-regulated, resulting in the down-regulation of Pax3 (paired box 3), and then inhibits cell proliferation, migration and invasion (Wang et al., 2018b), and triggers the occurrence of PE. HIF-1 can also regulate cell proliferation through non-coding RNAs. Under hypoxia, HIF-1 induces the up-regulation of miR-210-3p expression, reduces FGF1 (fibroblast growth factor 1) expression in selective intrauterine growth restriction, thereby inhibits the proliferation and invasion of trophoblast cell lines, resulting in the damage of the smaller placenta in twins (Li et al., 2019).
Hypoxia regulates cell autophagy
HIF-1 can regulate cell autophagy. In the ectopic endometrium of patients with endometriosis, HIF-1 and autophagy-related protein LC3 are highly expressed. HIF-1 promotes the migration and invasion of ESC by up-regulating autophagy pathway (Liu et al., 2017). Hypoxia can also regulate autophagy through non-coding RNA. For example, studies have shown that MiR-210 can regulate autophagy of endometriosis cells under hypoxia and participate in the pathogenesis of endometriosis: the increase of mir-210 under hypoxia leads to the decrease of Bcl-2 (B-cell lymphoma-2) expression, thereby reducing the combination of Bcl-2 and beclin-1, promoting autophagy of endometrium cells, and then promoting the pathological development of endometriosis (Xu et al., 2016).
HIF-1 can also regulate cell autophagy through non-coding RNAs. In endometriosis, hypoxia up-regulates HIF-1-mediated lncRNA-MALAT1 expression level in a dose-dependent manner. LncRNA-MALAT1 expression level is positively correlated with the expression levels of HIF-1 and autophagy marker LC3. The increased expression levels of HIF-1, Beclin1 and LC3B-II proteins lead to the activation of the autophagy pathway (Liu et al., 2019).
Hypoxia regulates cell differentiation
Hypoxia can regulate cell differentiation. Some studies have shown that hypoxia induces the occurrence of PE through differentiation pathway. Acute hypoxia leads to the up-regulation of PGHS-2 (prostaglandin H synthase 2) expression in trophoblast, leads to the increase of thromboxane and prostaglandin E2 levels, limits the differentiation of trophoblast, reduces the formation of syncytiotrophoblast, and leads to the occurrence of PE (Nelson et al., 1999). In another study, hypoxia reduces the expression of MAPK (mitogen-activated protein kinase)/ERK in BeWo cells and JEG3 cells, thereby down-regulates the expression of LIN28B (lin-28 homolog B) and SYN-1 (syncytin 1), inhibits cell differentiation, causes damage to syncytiotrophoblast formation, and promotes the development of PE (Canfield et al., 2019; Kudo et al., 2003).
Hypoxia can regulate cell differentiation through non-coding RNAs. Under hypoxia, the differentiation of primary human trophoblast cells is terminated, resulting in the down-regulation of miR-424 expression and up-regulation of FGFR1 (fibroblast growth factor receptor 1), leading to the occurrence of severe PE (Mouillet et al., 2013; Tang et al., 2019a). Hypoxia down-regulates NRF2 (nuclear factor erythroid 2-related factor 2), which is a redox sensitive transcription factor in trophoblast, thereby down-regulating miR-1246, aromatase, C/EBPβ (CCAAT/enhancer-binding protein beta) and PPARγ (peroxisome proliferator-activated receptor gamma). At the same time, the down-regulation of miR-1246 induces GSK3β (glycogen synthase kinase 3 beta) and AXIN2 expression, thereby inhibits the differentiation of syncytiotrophoblast, leading to the occurrence of PE (Muralimanoharan et al., 2018).
HIF-1 can directly regulate cell differentiation. Hypoxia promotes HIF-1 expression in human trophoblast cells, down-regulates the expression of ERRγ (estrogen-related receptor γ) and hCYP19, inhibits trophoblast differentiation, and leads to the occurrence of PE (Kumar & Mendelson, 2011). HIF-1 can also regulate cell differentiation through non-coding RNAs. Under hypoxia, HIF-1 is up-regulated, which induces the expression of miR-210, targets and inhibits CPEB2 (cytoplasmic polyadenylation element-binding 2), inhibits syncytialization of trophoblast cells, and leads to the occurrence of PE (Wang et al., 2020b).
Hypoxia regulates cell apoptosis
Hypoxia can regulate cell apoptosis. Studies have shown that PE may be related to excessive trophoblast apoptosis induced by hypoxia (Leung et al., 2001). Intermittent hypoxia can activate ER (endoplasmic reticulum) stress pathway, activate caspase-3 and PARP (poly(ADP-ribose) polymerase), up-regulate Bax (BCL-2-associated X) protein level, induce HTR-8/SVneo trophoblast cell apoptosis, inhibit invasion and migration, and eventually lead to the occurrence of PE (Song et al., 2020). Hypoxia up-regulates the expression level of p53 (Tan et al., 2019) and the cleaved caspase-9, activates trophoblast apoptosis, reduces the invasion ability of extravillous trophoblast, leads to abnormal remodeling of spiral artery, activates systemic inflammatory response (Sargent et al., 2003), and finally induces the occurrence of PE.
Hypoxia also regulates cell apoptosis through non-coding RNAs. Studies have shown that miR-141 in HTR-8/SVneo trophoblast cells targets CXCL12β (C–X–C motif chemokine ligand 12) in hypoxic environment, down-regulates MMP2, p62 and LC3B proteins, up-regulates ROCK1 (rho-associated kinase 1) and RhoA (ras homolog family member A) proteins, causes trophoblast apoptosis, inhibits trophoblast invasion and angiogenesis, thus causes the occurrence of PE (Wu et al., 2019). Under hypoxia, the up-regulation of miR-30b-5p in HTR-8/SVneo cells inhibits the expression of Pax3 and promotes cell apoptosis, thus leading to the occurrence of PE (Zhang et al., 2020a). Hypoxia up-regulates miR-141-3p in HUVECs (human umbilical vein endothelial cells), increases the expression of Bax and cleaved Caspase-3, down-regulates the expression of Notch2 (neurogenic locus notch homolog protein) and Bcl-2, promotes cell apoptosis, and inhibits the activity, tube formation, migration and invasion of HUVECs, thus leading to the occurrence of PE (Ling et al., 2021). Hypoxia can also reduce the expression of miR-19a-3p, increase the levels of lncRNA-PSG10P and IL1RAP (interleukin-1 receptor accessory protein), and lead to systemic inflammatory reaction in mother. The increased IL1RAP leads to the up-regulation of Caspase-3, which is the key apoptosis enzyme, promotes cell apoptosis and inhibits cell invasion and migration, and finally leads to the occurrence of PE (Wang et al., 2019).
HIF-1 can directly regulate cell apoptosis. FOXO3a (forkhead box O transcription factor 3a) regulates cell apoptosis (Brucker et al., 2016). Hypoxia activates HIF-1; and the up-regulation of FOXO3a increases trophoblast apoptosis and reduces its infiltration capacity, thus leading to the occurrence of PE (Zhang et al., 2020b).
Hypoxia regulates cell angiogenesis
HIF-1 can directly regulate angiogenesis. Endometriosis requires the generation of new blood vessels to supply nutrients (Taylor et al., 2002). Studies have shown that HIF-1 regulates the transcription of VEGF-A (vascular endothelial growth factor-A) and then regulates angiogenesis (Manalo et al., 2005). VEGF-A can stimulate angiogenesis and increase vascular permeability, so that the endometrium of women with endometriosis have higher angiogenesis potentiality (Carlo et al., 2009). Under hypoxia, HIF-1 overexpression can promote the expression of VEGF, PIGF (placental growth factor) and FLT-1 (fms related receptor tyrosine kinase 1), increase angiogenesis, and lead to the occurrence of PE (Rajakumar et al., 2004). Tyrosine kinase receptor 1 (FLT-1) is an angiogenic factor. Some studies have shown that hypoxia inhibits HIF-3α expression in HTR-8/SVneo cells, down-regulates FLT-1/JAK/STAT signaling pathway, promotes the apoptosis of extravillous cells, inhibits angiogenesis, and promotes the occurrence and development of PE (Qu, et al., 2021). HIF-1 can regulate angiogenesis through non-coding RNAs. The ectopic endometrium may initially develop ischemia and hypoxia, leading to the activation of HIF-1/VEGF signaling pathway. The overexpression of miR-199a can reduce the expression levels of HIF-1 and VEGF and then inhibit angiogenesis in endometriosis (Dai et al., 2015).
Hypoxia regulates the expression levels of estrogen receptors
ER (estrogen receiver) includes ERα (estrogen receptor α), ERβ (estrogen receiver β), and membrane receptor GPER (G protein-coupled estrogen receptor) (Fig. 3). ER is the key receptor to maintain the differentiation of ovarian granulosa cells, the growth and development of follicles and oocytes, and the functions of ovulation (Tang et al., 2019b). Dysfunctions of ER may result in the occurrence of female reproductive system diseases, such as breast cancer, endometriosis, endometrial cancer and ovarian cancer. Under hypoxia, HIF-1 down-regulates the protein level of ERα and suppresses its transcriptional activity in breast cancer cells, thereby producing ERα-negative phenotype and enhancing its resistance to endocrine therapy drugs (Cho et al., 2005; Whitman et al., 2019). In the other side, HIF-1 is also regulated by ERα. It has been reported that ERα/c-Src/PI3K/Akt/mTOR signaling pathway elevates the expression level of HIF-1 in response to oestrogen in ERα-contained breast cancer cells (Sudhagar et al., 2011). Different from ERα, ERβ inhibits the transcriptional activity of HIF-1 by down-regulating ARNT (aryl hydrocarbon receptor nuclear translocator), thereby inhibiting angiogenesis (Higashimura et al., 2016). GPER1 is a transmembrane G protein-coupled receptor that binds estrogen to activate multiple downstream signaling pathways (Prossnitz et al., 2008). E2 and GPER selective ligand (G-1) trigger the GPER signaling pathway, which leads to the up-regulation of HIF-1 and VEGF, and promotes the progression of breast cancer (Francesco et al., 2014). Therefore, estrogen receptors (ERα, ERβ and GPER) could crosstalk with HIF-1 (Fig. 3), indicating that hypoxia might regulate the ER-mediated the occurrence of female reproductive system diseases.
Fig. 3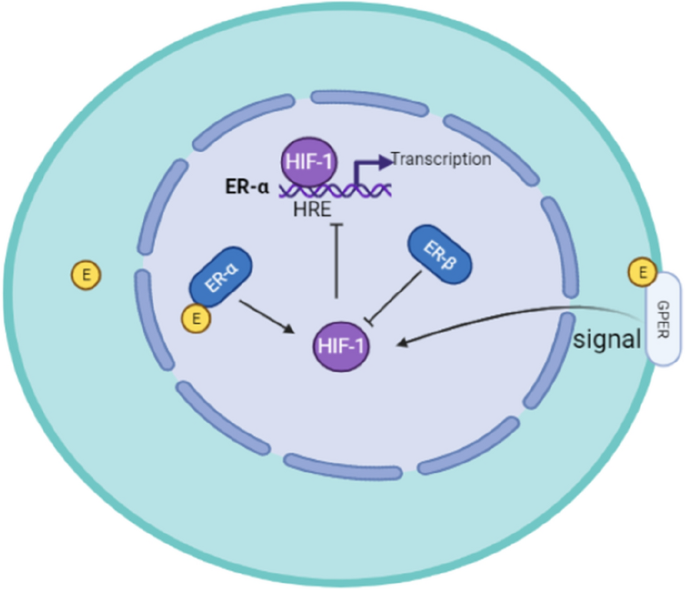
Crosstalk between HIF-1 and estrogen receptor (ER) in breast cancer cells
Full size imageConclusions
In conclusion, female reproductive problems are becoming increasingly prominent. The incidence rate and mortality rate of female tumor and non-tumor diseases are increasing year by year. Breast cancer has the leading incidence rate in the world. Ovarian cancer has become the major tumor threatening female life and health because of its high mortality, high recurrence rate, and high chemotherapy resistance. Cervical cancer has the first incidence rate among three major gynecological malignant tumors. The incidence rate and mortality of endometrial cancer are increasing with year and show a younger trend worldwide. For non-tumor diseases, endometriosis is the most common cause of chronic pelvic pain in women and is associated with infertility. The incidence of endometriosis is high in the reproductive period. Pregnancy-induced hypertension is one important reason for the increased maternal and perinatal mortality. FGR is often accompanied with various complications, including neonatal hypoxia, hypoglycemia, hyperbilirubinemia, sepsis and death. These diseases not only bring serious harm to women, but also threaten the development of our society.
As an important environmental factor, hypoxia is closely associated with female reproductive system diseases. Hypoxia induces cells to adapt to hypoxic microenvironment, which is an important link in trophoblast differentiation and placental formation. At the same time, hypoxia can also cause placental failure, leading to pathological changes, which produce adverse effects on female pregnancy and fetal health. HIFs (hypoxia inducible factors) can regulate the angiogenesis, glucose utilization, antioxidant stress, cell proliferation, anti-apoptotic, invasion and metastasis of trophoblast cells by regulating its downstream proteins (such as E-cadherin, Sox2, PLOD2, VEGF, etc.). In addition, HIF-1 is also closely associated with clinical stage, pathological grade, lymph node metastasis, recurrence, 5-year survival rate and other non-tumor pregnancy diseases. Therefore, HIF-1 is considered as an important indicator of early diagnosis, treatment effect and prognosis in female reproductive system diseases. HIF-1 inhibitors have been applied in clinical trials of several recurrent tumors, and are expected to be applied in other malignant tumors, which brings new expectations for the clinical treatment of female reproductive system diseases. However, the regulatory mechanisms still need to be further studied. The etiology of hypoxia in pregnant women is still unclear and should be further explored.
HIF-1 has an important regulatory role in female reproductive system diseases (Fig. 1). HIF-1 can up-regulate the expression of angiogenesis-related transcription factors (such as VEGFs, MMPs, ET-1 and ETA) and promote the formation of new blood vessels. HIF-1 can promote the expression of transcription factors related to cell proliferation (such as prostaglandin E receptor and PTGER4) and promote tumor cell proliferation through signal pathways (such as JNK/STAT5 and HIF-1/TGFA/EGFR/ErbB2/MYC). Moreover, HIF-1 can also promote the expression of transcription factors (such as TGF-α, PLOD2, Rab11, and MMP14), which promote tumor cell invasion and migration. In addition, HIF-1 up-regulates the levels of transcription factors related to cell invasion and migration (such as SNAIL, TWIST, ZEB1), which inhibit E-cadherin expression and promote EMT (epithelial mesenchymal transition) process. Furthermore, HIF-1 can also up-regulate autophagy-related transcription factors (such as IRE1, PERK, ATF4, Parkin), activate tumor cell autophagy, and promote tumor cell survival.
With the development of high-throughput sequencing, more and more studies have shown that miRNAs and lncRNAs play important role in regulating female reproductive system diseases caused by hypoxia. However, the breadth and depth of research are still very limited. There are still a large number of non-coding RNAs to be further undiscovered. Moreover, the regulatory mechanisms and networks of non-coding RNAs still need to be further studied. We hope to reveal their roles and regulatory mechanisms in the effect of hypoxia on female reproductive system diseases. In addition, it is also necessary to further identify reliable targets that can reflect hypoxia stress in female reproductive system diseases and to explore new approach for early detection and clinical treatment.
Data availability
Not applicable.
Abbreviations
ANTXR2:
Anthrax toxin receptor 2
ATF4:
Activating transcription factor 4
ARID1A:
AT-rich interaction domain 1A
ARNT:
Aryl hydrocarbon receptor nuclear translocator
Bax:
BCL-2-associated X
Bcl-2:
B-cell lymphoma-2
BCL6:
B-cell lymphoma 6 protein
BNIP3:
Bcl-2 interacting protein 3
CCL17:
Chemokine ligand 17
C/EBPβ:
CCAAT/enhancer-binding protein beta
COUP-TFII:
Chicken ovalbumin upstream-transcription factor II
COX-2:
Cyclooxygenase-2
CPEB2:
Cytoplasmic polyadenylation element-binding 2
CXCL12β:
C–X–C motif chemokine ligand 12
CYR61:
Cysteine-rich angiogenic inducer 61
DDX3:
DEAD-box helicase 3 X-linked
DUSP2:
Dual-specificity phosphatase-2
E2:
Estrogen 2
EG-VEGF :
Endocrine gland-derived vascular endothelial growth factor
EMT:
Epithelial–mesenchymal transition
EPO:
Erythropoietin
ER:
Endoplasmic reticulum
ER:
Estrogen receiver
ERα:
Estrogen receptor α
ERβ:
Estrogen receiver β
ERK:
Extracellular signal-regulated kinase
ERRγ:
Estrogen-related receptor γ
ESC:
Endometrial stromal cells
ET-1:
Endothelin-1
ETAR:
Endothelin A receptor
FGF1:
Fibroblast growth factor 1
FGR:
Fetal growth restriction
FGFR1:
Fibroblast growth factor receptor 1
FLT-1:
FMS-like tyrosine kinase 1
FOXO3a:
Forkhead box O transcription factor 3a
GSK3β:
Glycogen synthase kinase 3 beta
GPER:
G protein-coupled estrogen receptor
HIF-1:
Hypoxia-inducible factor-1
HIFs:
Hypoxia-inducible factors
HK2:
Hexokinase II
HuR:
Human antigen R
HUVECs:
Human umbilical vein endothelial cells
HDAC6:
Histone deacetylase 6
IGFBP-1:
Insulin-like growth factor binding protein-1
IL-6:
Interleukin-6
IL1RAP:
Interleukin-1 receptor accessory protein
IR:
Ionizing radiation
IRE1:
Inositol required protein 1
JNK:
Janus kinase
KRAS:
KRAS proto-oncogene, GTPase
lncRNA-MALAT1:
Long non-coding RNA malat 1
LIN28B:
Lin-28 homolog B
MAPK:
Mitogen-activated protein kinase
MMP14:
Matrix metalloproteinase 14
mTOR:
The mammalian target of rapamycin
NRF2:
Nuclear factor erythroid 2-related factor 2
Notch2:
Neurogenic locus notch homolog protein
OCCC:
Ovarian clear cell carcinoma
PARP:
Poly(ADP-ribose) polymerase
PDK1:
Pyruvate dehydrogenase kinase 1
PE:
Preeclampsia
PERK:
Protein kinase RNA-like ER kinase
PGE2:
Prostaglandin E2
PGHS-2:
Prostaglandin H synthase 2
PIGF:
Placental growth factor
PLOD2:
Procollagen lysine, 2-oxoglutarate, 5-dioxygenase
PO2 :
Oxygen partial pressure
PPARγ :
Peroxisome proliferator-activated receptor gamma
PTBP3:
Polypyrimidine binding protein 3
PTGER4:
Prostaglandin e receptor 4
RhoA:
Ras homolog family member A
ROCK1:
Rho-associated kinase 1
ROS:
Reactive oxygen species
SNAIL:
Snail family transcriptional repressor
STAT:
Signal transducer and activator of transcription
SYN-1:
Syncytin 1
TAM:
Tumor-associated macrophages
TCF3:
Transcription factor 3
TGF-β:
Transforming growth factor β
TIC:
Tumor-initiating cells
TNBC:
Three negative breast cancer
TWIST:
Twist family bHLH transcription factor
uPAR:
Urokinase receptor
VEGF:
Vascular endothelial growth factor
YAP1:
Yes-associated protein 1
ZEB1:
Zinc finger E-box-binding homeobox 1
References
Akhilesh, M., Mahalingam, V., Nalliah, S., Ali, R. M., Ganesalingam, M., & Haleagrahara, N. (2013). Hypoxia-inducible factor-1alpha as a predictive marker in pre-eclampsia. Biomed Rep, 1(2), 257–258.
Bensaad, K., & Harris, A. L. (2014). Hypoxia and metabolism in cancer. Advances in Experimental Medicine and Biology, 772, 1–39.
Bol, G. M., Raman, V., van der Groep, P., Vermeulen, J. F., Patel, A. H., van der Wall, E., & van Diest, P. J. (2013). Expression of the RNA helicase DDX3 and the hypoxia response in breast cancer. PLoS One, 8(5), e63548.
Brucker, D. P., Maurer, G. D., Harter, P. N., Rieger, J., & Steinbach, J. P. (2016). FOXO3a orchestrates glioma cell responses to starvation conditions and promotes hypoxia-induced cell death. International Journal of Oncology, 49(6), 2399–2410.
Canfield, J., Arlier, S., Mong, E. F., Lockhart, J., VanWye, J., Guzeloglu-Kayisli, O., Schatz, F., Magness, R. R., Lockwood, C. J., Tsibris, J. C. M., Kayisli, U. A., & Totary-Jain, H. (2019). Decreased LIN28B in preeclampsia impairs human trophoblast differentiation and migration. The FASEB Journal, 33(2), 2759–2769.
Cao, L., Wang, M., Dong, Y., Xu, B., Chen, J., Ding, Y., Qiu, S., Li, L., Karamfilova Zaharieva, E., Zhou, X., & Xu, Y. (2020). Circular RNA circRNF20 promotes breast cancer tumorigenesis and Warburg effect through miR-487a/HIF-1alpha/HK2. Cell Death & Disease, 11(2), 145.
Catalano, R. D., Wilson, M. R., Boddy, S. C., McKinlay, A. T., Sales, K. J., & Jabbour, H. N. (2011). Hypoxia and prostaglandin E receptor 4 signalling pathways synergise to promote endometrial adenocarcinoma cell proliferation and tumour growth. PLoS One, 6(5), e19209.
Chen, G., Xu, X., Zhang, L., Fu, Y., Wang, M., Gu, H., & Xie, X. (2014). Blocking autocrine VEGF signaling by sunitinib, an anti-cancer drug, promotes embryonic stem cell self-renewal and somatic cell reprogramming. Cell Research, 24(9), 1121–1136.
Chen, G., Liu, B., Yin, S., Li, S., Guo, Y., Wang, M., Wang, K., & Wan, X. (2020a). Hypoxia induces an endometrial cancer stem-like cell phenotype via HIF-dependent demethylation of SOX2 mRNA. Oncogenesis, 9(9), 81.
Chen, P. S., Chiu, W. T., Hsu, P. L., Lin, S. C., Peng, I. C., Wang, C. Y., & Tsai, S. J. (2020b). Pathophysiological implications of hypoxia in human diseases. Journal of Biomedical Science, 27(1), 63.
Cheng, K. W., Lahad, J. P., Kuo, W. L., Lapuk, A., Yamada, K., Auersperg, N., Liu, J., Smith-McCune, K., Lu, K. H., Fishman, D., Gray, J. W., & Mills, G. B. (2004). The RAB25 small GTPase determines aggressiveness of ovarian and breast cancers. Nature Medicine, 10(11), 1251–1256.
Cho, J., Kim, D., Lee, S., & Lee, Y. (2005). Cobalt chloride-induced estrogen receptor alpha down-regulation involves hypoxia-inducible factor-1alpha in MCF-7 human breast cancer cells. Molecular Endocrinology, 19(5), 1191–1199.
Cianfrocca, R., Tocci, P., Rosanò, L., Caprara, V., Sestito, R., Di Castro, V., & Bagnato, A. (2016). Nuclear beta-arrestin1 is a critical cofactor of hypoxia-inducible factor-1alpha signaling in endothelin-1-induced ovarian tumor progression. Oncotarget, 7(14), 17790–17804.
Dai, L., Lou, W., Zhu, J., Zhou, X., & Di, W. (2015). MiR-199a inhibits the angiogenic potential of endometrial stromal cells under hypoxia by targeting HIF-1alpha/VEGF pathway. International Journal of Clinical and Experimental Pathology, 8(5), 4735–4744.
Davis, F. M., Azimi, I., Faville, R. A., Peters, A. A., Jalink, K., Putney, J. W., Jr., Goodhill, G. J., Thompson, E. W., Roberts-Thomson, S. J., & Monteith, G. R. (2014). Induction of epithelial-mesenchymal transition (EMT) in breast cancer cells is calcium signal dependent. Oncogene, 33(18), 2307–2316.
De Francesco, E. M., Pellegrino, M., Santolla, M. F., Lappano, R., Ricchio, E., Abonante, S., & Maggiolini, M. (2014). GPER mediates activation of HIF1alpha/VEGF signaling by estrogens. Cancer Research, 74(15), 4053–4064.
de Grauw, T. J., Myers, R. E., & Scott, W. J. (1986). Fetal growth retardation in rats from different levels of hypoxia. Biology of the Neonate, 49(2), 85–89.
Di Carlo, C., Bonifacio, M., Tommaselli, G. A., Bifulco, G., Guerra, G., & Nappi, C. (2009). Metalloproteinases, vascular endothelial growth factor, and angiopoietin 1 and 2 in eutopic and ectopic endometrium. Fertility and Sterility, 91(6), 2315–2323.
Fruehauf, J. P., & Meyskens, F. L., Jr. (2007). Reactive oxygen species: A breath of life or death? Clinical Cancer Research, 13(3), 789–794.
George, E. M., Garrett, M. R., & Granger, J. P. (2014). Placental ischemia induces changes in gene expression in chorionic tissue. Mammalian Genome, 25(5–6), 253–261.
Harris, A. L. (2002). Hypoxia–a key regulatory factor in tumour growth. Nature Reviews Cancer, 2(1), 38–47.
Higashimura, Y., Kitakaze, T., Harada, N., Inui, H., Nakano, Y., & Yamaji, R. (2016). pVHL-mediated degradation of HIF-2alpha regulates estrogen receptor alpha expression in normoxic breast cancer cells. FEBS Letters, 590(16), 2690–2699.
Hillebrand, L. E., Wickberg, S. M., Gomez-Auli, A., Follo, M., Maurer, J., Busch, H., Boerries, M., & Reinheckel, T. (2019). MMP14 empowers tumor-initiating breast cancer cells under hypoxic nutrient-depleted conditions. The FASEB Journal, 33(3), 4124–4140.
Hofbauer, K. H., Gess, B., Lohaus, C., Meyer, H. E., Katschinski, D., & Kurtz, A. (2003). Oxygen tension regulates the expression of a group of procollagen hydroxylases. European Journal of Biochemistry, 270(22), 4515–4522.
Hohenberger, P., Felgner, C., Haensch, W., & Schlag, P. M. (1998). Tumor oxygenation correlates with molecular growth determinants in breast cancer. Breast Cancer Research and Treatment, 48(2), 97–106.
Hsiao, K. Y., Chang, N., Tsai, J. L., Lin, S. C., Tsai, S. J., & Wu, M. H. (2017). Hypoxia-inhibited DUSP2 expression promotes IL-6/STAT3 signaling in endometriosis. American Journal of Reproductive Immunology, 78(4), e16290.
Ishikawa, H., Xu, L., Sone, K., Kobayashi, T., Wang, G., & Shozu, M. (2019). Hypoxia induces hypoxia-inducible factor 1alpha and potential HIF-responsive gene expression in uterine leiomyoma. Reproductive Sciences, 26(3), 428–435.
Jo, M., Lester, R. D., Montel, V., Eastman, B., Takimoto, S., & Gonias, S. L. (2009). Reversibility of epithelial-mesenchymal transition (EMT) induced in breast cancer cells by activation of urokinase receptor-dependent cell signaling. Journal of Biological Chemistry, 284(34), 22825–22833.
Kaelin, W. G., Jr., & Ratcliffe, P. J. (2008). Oxygen sensing by metazoans: The central role of the HIF hydroxylase pathway. Molecular Cell, 30(4), 393–402.
Kinose, Y., Sawada, K., Nakamura, K., Sawada, I., Toda, A., Nakatsuka, E., Hashimoto, K., Mabuchi, S., Takahashi, K., Kurachi, H., Lengyel, E., & Kimura, T. (2015). The hypoxia-related microRNA miR-199a-3p displays tumor suppressor functions in ovarian carcinoma. Oncotarget, 6(13), 11342–11356.
Krishnamachary, B., Zagzag, D., Nagasawa, H., Rainey, K., Okuyama, H., Baek, J. H., & Semenza, G. L. (2006). Hypoxia-inducible factor-1-dependent repression of E-cadherin in von Hippel-Lindau tumor suppressor-null renal cell carcinoma mediated by TCF3, ZFHX1A, and ZFHX1B. Cancer Research, 66(5), 2725–2731.
Kudo, Y., Boyd, C. A., Sargent, I. L., & Redman, C. W. (2003). Hypoxia alters expression and function of syncytin and its receptor during trophoblast cell fusion of human placental BeWo cells: Implications for impaired trophoblast syncytialisation in pre-eclampsia. Biochimica Et Biophysica Acta, 1638(1), 63–71.
Kumar, P., & Mendelson, C. R. (2011). Estrogen-related receptor gamma (ERRgamma) mediates oxygen-dependent induction of aromatase (CYP19) gene expression during human trophoblast differentiation. Molecular Endocrinology, 25(9), 1513–1526.
Kunz, M., & Ibrahim, S. M. (2003). Molecular responses to hypoxia in tumor cells. Molecular Cancer, 2, 23.
Leung, D. N., Smith, S. C., Toh, K. F., Sahota, D. S., & Baker, P. N. (2001). Increased placental apoptosis in pregnancies complicated by preeclampsia. American Journal of Obstetrics and Gynecology, 184(6), 1249–1250.
Li, L., Huang, X., He, Z., Xiong, Y., & Fang, Q. (2019). miRNA-210-3p regulates trophoblast proliferation and invasiveness through fibroblast growth factor 1 in selective intrauterine growth restriction. Journal of Cellular and Molecular Medicine, 23(6), 4422–4433.
Li, F., Qiao, Z., Duan, Q., & Nevo, E. (2021). Adaptation of mammals to hypoxia. Animal Models and Experimental Medicine, 4(4), 311–318.
Liang, Y., Song, X., Li, Y., Chen, B., Zhao, W., Wang, L., Zhang, H., Liu, Y., Han, D., Zhang, N., Ma, T., Wang, Y., Ye, F., Luo, D., Li, X., & Yang, Q. (2020). LncRNA BCRT1 promotes breast cancer progression by targeting miR-1303/PTBP3 axis. Molecular Cancer, 19(1), 85.
Lin, S. C., Wang, C. C., Wu, M. H., Yang, S. H., Li, Y. H., & Tsai, S. J. (2012). Hypoxia-induced microRNA-20a expression increases ERK phosphorylation and angiogenic gene expression in endometriotic stromal cells. Journal of Clinical Endocrinology and Metabolism, 97(8), E1515–E1523.
Ling, Z., Chen, M., Li, T., Qian, Y., & Li, C. (2021). MiR-141-3p downregulation promotes tube formation, migration, invasion and inhibits apoptosis in hypoxia-induced human umbilical vein endothelial cells by targeting Notch2. Reproductive Biology, 21(2), 100483.
Liu, L. B., Xie, F., Chang, K. K., Shang, W. Q., Meng, Y. H., Yu, J. J., Li, H., Sun, Q., Yuan, M. M., Jin, L. P., Li, D. J., & Li, M. Q. (2015). Chemokine CCL17 induced by hypoxia promotes the proliferation of cervical cancer cell. American Journal of Cancer Research, 5(10), 3072–3084.
Liu, H., Zhang, Z., Xiong, W., Zhang, L., Xiong, Y., Li, N., He, H., Du, Y., & Liu, Y. (2017). Hypoxia-inducible factor-1alpha promotes endometrial stromal cells migration and invasion by upregulating autophagy in endometriosis. Reproduction, 153(6), 809–820.
Liu, H., Zhang, Z., Xiong, W., Zhang, L., Du, Y., Liu, Y., & Xiong, X. (2019). Long non-coding RNA MALAT1 mediates hypoxia-induced pro-survival autophagy of endometrial stromal cells in endometriosis. Journal of Cellular and Molecular Medicine, 23(1), 439–452.
Manalo, D. J., Rowan, A., Lavoie, T., Natarajan, L., Kelly, B. D., Ye, S. Q., Garcia, J. G., & Semenza, G. L. (2005). Transcriptional regulation of vascular endothelial cell responses to hypoxia by HIF-1. Blood, 105(2), 659–669.
Matouk, I. J., Raveh, E., Abu-lail, R., Mezan, S., Gilon, M., Gershtain, E., Birman, T., Gallula, J., Schneider, T., Barkali, M., Richler, C., Fellig, Y., Sorin, V., Hubert, A., Hochberg, A., & Czerniak, A. (2014). Oncofetal H19 RNA promotes tumor metastasis. Biochimica Et Biophysica Acta, 1843(7), 1414–1426.
Meeker, J. D., Sathyanarayana, S., & Swan, S. H. (2009). Phthalates and other additives in plastics: Human exposure and associated health outcomes. Philosophical Transactions of the Royal Society of London. Series B, Biological Sciences, 364(1526), 2097–2113.
Meroni, S. B., Galardo, M. N., Rindone, G., Gorga, A., Riera, M. F., & Cigorraga, S. B. (2013). Uterine fibroids are characterized by an impaired antioxidant cellular system: Potential role of hypoxia in the pathophysiology of uterine fibroids. Journal of Assisted Reproduction and Genetics, 30(7), 969–974.
Meroni, S. B., Galardo, M. N., Rindone, G., Gorga, A., Riera, M. F., & Cigorraga, S. B. (2019). Molecular mechanisms and signaling pathways involved in sertoli cell proliferation. Frontiers in Endocrinology (Lausanne), 10, 224.
Moreno-Bueno, G., Portillo, F., & Cano, A. (2008). Transcriptional regulation of cell polarity in EMT and cancer. Oncogene, 27(55), 6958–6969.
Mouillet, J. F., Donker, R. B., Mishima, T., Cronqvist, T., Chu, T., & Sadovsky, Y. (2013). The unique expression and function of miR-424 in human placental trophoblasts. Biology of Reproduction, 89(2), 25.
Muralimanoharan, S., Kwak, Y. T., & Mendelson, C. R. (2018). Redox-sensitive transcription factor NRF2 enhances trophoblast differentiation via induction of miR-1246 and aromatase. Endocrinology, 159(5), 2022–2033.
Nagpal, N., Ahmad, H. M., Chameettachal, S., Sundar, D., Ghosh, S., & Kulshreshtha, R. (2015). HIF-inducible miR-191 promotes migration in breast cancer through complex regulation of TGFbeta-signaling in hypoxic microenvironment. Science and Reports, 5, 9650.
Nelson, D. M., Johnson, R. D., Smith, S. D., Anteby, E. Y., & Sadovsky, Y. (1999). Hypoxia limits differentiation and up-regulates expression and activity of prostaglandin H synthase 2 in cultured trophoblast from term human placenta. American Journal of Obstetrics and Gynecology, 180(4), 896–902.
Notte, A., Rebucci, M., Fransolet, M., Roegiers, E., Genin, M., Tellier, C., Watillon, K., Fattaccioli, A., Arnould, T., & Michiels, C. (2015). Taxol-induced unfolded protein response activation in breast cancer cells exposed to hypoxia: ATF4 activation regulates autophagy and inhibits apoptosis. International Journal of Biochemistry & Cell Biology, 62, 1–14.
Petousi, N., & Robbins, P. A. (2014). Human adaptation to the hypoxia of high altitude: The Tibetan paradigm from the pregenomic to the postgenomic era. Journal of Applied Physiology (1985), 116(7), 875–884.
Prossnitz, E. R., Arterburn, J. B., Smith, H. O., Oprea, T. I., Sklar, L. A., & Hathaway, H. J. (2008). Estrogen signaling through the transmembrane G protein-coupled receptor GPR30. Annual Review of Physiology, 70, 165–190.
Qiu, Z., Wang, L., & Liu, H. (2021). Hsa_circ_0001982 promotes the progression of breast cancer through miR-1287-5p/MUC19 axis under hypoxia. World Journal of Surgical Oncology, 19(1), 161.
Qu, H., Yu, Q., Jia, B., Zhou, W., Zhang, Y., & Mu, L. (2021). HIF3alpha affects preeclampsia development by regulating EVT growth via activation of the Flt1/JAK/STAT signaling pathway in hypoxia. Molecular Medicine Reports, 23(1), 68.
Rajakumar, A., Brandon, H. M., Daftary, A., Ness, R., & Conrad, K. P. (2004). Evidence for the functional activity of hypoxia-inducible transcription factors overexpressed in preeclamptic placentae. Placenta, 25(10), 763–769.
Rath, G., Aggarwal, R., Jawanjal, P., Tripathi, R., & Batra, A. (2016). HIF-1 alpha and placental growth factor in pregnancies complicated with preeclampsia: A qualitative and quantitative analysis. Journal of Clinical Laboratory Analysis, 30(1), 75–83.
Ream, M., Ray, A. M., Chandra, R., & Chikaraishi, D. M. (2008). Early fetal hypoxia leads to growth restriction and myocardial thinning. American Journal of Physiology-Regulatory, Integrative and Comparative Physiology, 295(2), R583–R595.
Sargent, I. L., Germain, S. J., Sacks, G. P., Kumar, S., & Redman, C. W. (2003). Trophoblast deportation and the maternal inflammatory response in pre-eclampsia. Journal of Reproductive Immunology, 59(2), 153–160.
Schindl, M., Schoppmann, S. F., Samonigg, H., Hausmaninger, H., Kwasny, W., Gnant, M., Jakesz, R., Kubista, E., Birner, P., Oberhuber, G., Austrian Breast and Colorectal Cancer Study Group. (2002). Overexpression of hypoxia-inducible factor 1alpha is associated with an unfavorable prognosis in lymph node-positive breast cancer. Clinical Cancer Research, 8(6), 1831–1837.
Selvendiran, K., Bratasz, A., Kuppusamy, M. L., Tazi, M. F., Rivera, B. K., & Kuppusamy, P. (2009). Hypoxia induces chemoresistance in ovarian cancer cells by activation of signal transducer and activator of transcription 3. International Journal of Cancer, 125(9), 2198–2204.
Shields, T., Gridley, G., Moradi, T., Adami, J., Plato, N., & Dosemeci, M. (2002). Occupational exposures and the risk of ovarian cancer in Sweden. American Journal of Industrial Medicine, 42(3), 200–213.
Sivridis, E., Giatromanolaki, A., Gatter, K. C., Harris, A. L., Koukourakis, M. I., Tumor and Angiogenesis Research Group. (2002). Association of hypoxia-inducible factors 1alpha and 2alpha with activated angiogenic pathways and prognosis in patients with endometrial carcinoma. Cancer, 95(5), 1055–1063.
Song, L., Liu, S., Zhang, L., Yao, H., Gao, F., Xu, D., & Li, Q. (2016). MiR-21 modulates radiosensitivity of cervical cancer through inhibiting autophagy via the PTEN/Akt/HIF-1alpha feedback loop and the Akt-mTOR signaling pathway. Tumour Biology, 37(9), 12161–12168.
Song, W., Chang, W. L., Shan, D., Gu, Y., Gao, L., Liang, S., Guo, H., Yu, J., & Liu, X. (2020). Intermittent hypoxia impairs trophoblast cell viability by triggering the endoplasmic reticulum stress pathway. Reproductive Sciences, 27(2), 477–487.
Sudhagar, S., Sathya, S., & Lakshmi, B. S. (2011). Rapid non-genomic signalling by 17beta-oestradiol through c-Src involves mTOR-dependent expression of HIF-1alpha in breast cancer cells. British Journal of Cancer, 105(7), 953–960.
Taguchi, Y., Horiuchi, Y., Kano, F., & Murata, M. (2017). Novel prosurvival function of Yip1A in human cervical cancer cells: Constitutive activation of the IRE1 and PERK pathways of the unfolded protein response. Cell Death & Disease, 8(3), e2718.
Tan, B., Tong, C., Yuan, Y., Xu, P., Wen, L., Zhang, C., Zheng, Y., Lin, L., Zhu, F., Gui, S., Wang, L., Gao, R., Li, J., Qi, H., & Baker, P. N. (2019). The regulation of trophoblastic p53 homeostasis by the p38-Wip1 feedback loop is disturbed in placentas from pregnancies complicated by preeclampsia. Cellular Physiology and Biochemistry, 52(2), 315–335.
Tanfin, Z., Leiber, D., Robin, P., Oyeniran, C., & Breuiller-Fouché, M. (2011). Endothelin-1: Physiological and pathological roles in myometrium. International Journal of Biochemistry & Cell Biology, 43(3), 299–302.
Tanfin, Z., & Breuiller-Fouche, M. (2012). The endothelin axis in uterine leiomyomas: New insights. Biology of Reproduction, 87(1), 5–10.
Tang, X. L., Lin, L., Song, L. N., & Tang, X. H. (2016). Hypoxia-inducible miR-152 suppresses the expression of WNT1 and ERBB3, and inhibits the proliferation of cervical cancer cells. Experimental Biology and Medicine (Maywood, N.J.), 241(13), 1429–1437.
Tang, Q., Gui, J., Wu, X., & Wu, W. (2019a). Downregulation of miR-424 in placenta is associated with severe preeclampsia. Pregnancy Hypertension, 17, 109–112.
Tang, Z. R., Zhang, R., Lian, Z. X., Deng, S. L., & Yu, K. (2019b). Estrogen-receptor expression and function in female reproductive disease. Cells, 8(10), 1123.
Tang, K., Zhu, L., Chen, J., Wang, D., Zeng, L., Chen, C., Tang, L., Zhou, L., Wei, K., Zhou, Y., Lv, J., Liu, Y., Zhang, H., Ma, J., & Huang, B. (2021). Hypoxia promotes breast cancer cell growth by activating a glycogen metabolic program. Cancer Research, 81(19), 4949–4963.
Taylor, R. N., Lebovic, D. I., & Mueller, M. D. (2002). Angiogenic factors in endometriosis. Annals of the New York Academy of Sciences, 955, 89–100. discussion 118, 396-406.
Vaupel, P., Hockel, M., & Mayer, A. (2007). Detection and characterization of tumor hypoxia using pO2 histography. Antioxidants & Redox Signaling, 9(8), 1221–1235.
Wallace, K., Chatman, K., Porter, J., Scott, J., Johnson, V., Moseley, J., & LaMarca, B. (2014). Enodthelin 1 is elevated in plasma and explants from patients having uterine leiomyomas. Reproductive Sciences, 21(9), 1196–1205.
Wallace, K., Chatman, K., Johnson, V., Brookins, A., Rushing, J., & LaMarca, B. (2018). Novel treatment avenues for uterine leiomyoma: A new implication for endothelin? Clinical Science (London, England), 132(20), 2261–2267.
Wang, Y., & Liu, S. (2021). LncRNA GHET1 promotes hypoxia-induced glycolysis, proliferation, and invasion in triple-negative breast cancer through the hippo/YAP signaling pathway. Frontiers in Cell and Developmental Biology, 9, 643515.
Wang, X. H., He, X., Jin, H. Y., Liang, J. X., & Li, N. (2018a). Effect of hypoxia on the Twist1 in EMT of cervical cancer cells. European Review for Medical and Pharmacological Sciences, 22(20), 6633–6639.
Wang, N., Feng, Y., Xu, J., Zou, J., Chen, M., He, Y., Liu, H., Xue, M., & Gu, Y. (2018b). miR-362-3p regulates cell proliferation, migration and invasion of trophoblastic cells under hypoxia through targeting Pax3. Biomedicine & Pharmacotherapy, 99, 462–468.
Wang, N., Li, R., & Xue, M. (2019). Potential regulatory network in the PSG10P/miR-19a-3p/IL1RAP pathway is possibly involved in preeclampsia pathogenesis. Journal of Cellular and Molecular Medicine, 23(2), 852–864.
Wang, L., Li, Y. S., Yu, L. G., Zhang, X. K., Zhao, L., Gong, F. L., Yang, X. X., & Guo, X. L. (2020a). Galectin-3 expression and secretion by tumor-associated macrophages in hypoxia promotes breast cancer progression. Biochemical Pharmacology, 178, 114113.
Wang, H., Zhao, Y., Luo, R., Bian, X., Wang, Y., Shao, X., Li, Y. X., Liu, M., & Wang, Y. L. (2020b). A positive feedback self-regulatory loop between miR-210 and HIF-1alpha mediated by CPEB2 is involved in trophoblast syncytialization: Implication of trophoblast malfunction in preeclampsiadagger. Biology of Reproduction, 102(3), 560–570.
Whitman, N. A., Lin, Z. W., Kenney, R. M., Albertini, L., & Lockett, M. R. (2019). Hypoxia differentially regulates estrogen receptor alpha in 2D and 3D culture formats. Archives of Biochemistry and Biophysics, 671, 8–17.
Wu, M. H., Chen, K. F., Lin, S. C., Lgu, C. W., & Tsai, S. J. (2007). Aberrant expression of leptin in human endometriotic stromal cells is induced by elevated levels of hypoxia inducible factor-1alpha. American Journal of Pathology, 170(2), 590–598.
Wu, D., Chen, X., Wang, L., Chen, F., Cen, H., & Shi, L. (2019). Hypoxia-induced microRNA-141 regulates trophoblast apoptosis, invasion, and vascularization by blocking CXCL12beta/CXCR2/4 signal transduction. Biomedicine & Pharmacotherapy, 116, 108836.
Xing, F., Okuda, H., Watabe, M., Kobayashi, A., Pai, S. K., Liu, W., Pandey, P. R., Fukuda, K., Hirota, S., Sugai, T., Wakabayshi, G., Koeda, K., Kashiwaba, M., Suzuki, K., Chiba, T., Endo, M., Mo, Y. Y., & Watabe, K. (2011). Hypoxia-induced Jagged2 promotes breast cancer metastasis and self-renewal of cancer stem-like cells. Oncogene, 30(39), 4075–4086.
Xu, T. X., Zhao, S. Z., Dong, M., & Yu, X. R. (2016). Hypoxia responsive miR-210 promotes cell survival and autophagy of endometriotic cells in hypoxia. European Review for Medical and Pharmacological Sciences, 20(3), 399–406.
Xu, H., Yuan, Y., Wu, W., Zhou, M., Jiang, Q., Niu, L., Ji, J., Liu, N., Zhang, L., & Wang, X. (2017). Hypoxia stimulates invasion and migration of human cervical cancer cell lines HeLa/SiHa through the Rab11 trafficking of integrin alphavbeta3/FAK/PI3K pathway-mediated Rac1 activation. Journal of Biosciences, 42(3), 491–499.
Xu, C., Zhu, L. X., Sun, D. M., Yao, H., & Han, D. X. (2020). Regulatory mechanism of lncRNA NORAD on proliferation and invasion of ovarian cancer cells through miR-199a-3p. European Review for Medical and Pharmacological Sciences, 24(4), 1672–1681.
Zamudio, S. (2003). The placenta at high altitude. High Altitude Medicine & Biology, 4(2), 171–191.
Zhang, Y., Fan, N., & Yang, J. (2015). Expression and clinical significance of hypoxia-inducible factor 1alpha, snail and E-cadherin in human ovarian cancer cell lines. Molecular Medicine Reports, 12(3), 3393–3399.
Zhang, K., Kong, X., Feng, G., Xiang, W., Chen, L., Yang, F., Cao, C., Ding, Y., Chen, H., Chu, M., Wang, P., & Zhang, B. (2018). Investigation of hypoxia networks in ovarian cancer via bioinformatics analysis. Journal of Ovarian Research, 11(1), 16.
Zhang, H., He, Y., Wang, J. X., Chen, M. H., Xu, J. J., Jiang, M. H., Feng, Y. L., & Gu, Y. F. (2020a). miR-30-5p-mediated ferroptosis of trophoblasts is implicated in the pathogenesis of preeclampsia. Redox Biology, 29, 101402.
Zhang, Z., Huang, C., Wang, P., Gao, J., Liu, X., Li, Y., Yan, S., & Shi, Y. (2020b). HIF1alpha affects trophoblastic apoptosis involved in the onset of preeclampsia by regulating FOXO3a under hypoxic conditions. Molecular Medicine Reports, 21(6), 2484–2492.
Zheng, R., Yao, Q., Xie, G., Du, S., Ren, C., Wang, Y., & Yuan, Y. (2015). TAT-ODD-p53 enhances the radiosensitivity of hypoxic breast cancer cells by inhibiting Parkin-mediated mitophagy. Oncotarget, 6(19), 17417–17429.
Zhong, R., Xu, H., Chen, G., Zhao, G., Gao, Y., Liu, X., Ma, S., & Dong, L. (2015). The role of hypoxia-inducible factor-1alpha in radiation-induced autophagic cell death in breast cancer cells. Tumour Biology, 36(9), 7077–7083.
Zhong, R., Xu, H., Chen, G., Zhao, G., Gao, Y., Liu, X., Ma, S., & Dong, L. (2019). Impairment of HIF-1alpha-mediated metabolic adaption by NRF2-silencing in breast cancer cells. Redox Biology, 24, 101210.
Acknowledgements
The authors acknowledge financial support from the Natural Science Foundation of China (NSFC No. 82073589), Shenzhen Science and Technology Program (No. JCYJ20210324114814038), Futian Healthcare Research Project (No. FTWS2021009), high-level talent project funds of Fujian Medical University (No. XRCZX2020030), and the Research Funds from the Eighth Affiliated Hospital of Sun Yat-sen University.
Funding
Not applicable.
Author information
Chenglong Ma, Zhaodian Zheng, Yanqiu Shen and Jing Fang have contributed equally to this work.
Authors and Affiliations
Key Laboratory of Environment and Female Reproductive Health, The Eighth Affiliated Hospital, Sun Yat-Sen University, Shenzhen, 518033, China
Chenglong Ma, Zhaodian Zheng, Yanqiu Shen, Jing Fang, Xinying Huang, Jiarong Guo & Huidong Zhang
Department of Toxicology, School of Public Health, Fujian Medical University, Fuzhou, 350122, China
Chenglong Ma, Zhaodian Zheng, Yanqiu Shen, Jing Fang & Xinying Huang
Contributions
CM, ZZ, YS, JF, and HZ conceived the project and wrote the paper. CM and HZ were involved in the critical revision of the manuscript. XH and JG contributed to collect information. All authors read and approved the final manuscript.
Corresponding author
Correspondence to Huidong Zhang.
Ethics declarations
Conflict of interest
The authors declare that they have no competing interests.
Ethical approval
Not applicable.
Consent to participate
Not applicable.
Consent for publication
Not applicable.
Rights and permissions
Springer Nature or its licensor holds exclusive rights to this article under a publishing agreement with the author(s) or other rightsholder(s); author self-archiving of the accepted manuscript version of this article is solely governed by the terms of such publishing agreement and applicable law.
About this article
Cite this article
Ma, C., Zheng, Z., Shen, Y. et al. The effects of hypoxia on female reproductive system diseases. GENOME INSTAB. DIS. (2022). https://doi.org/10.1007/s42764-022-00081-2
Received10 May 2022
Revised13 July 2022
Accepted26 August 2022
Published10 October 2022
DOIhttps://doi.org/10.1007/s42764-022-00081-2
Share this article
Anyone you share the following link with will be able to read this content:
Get shareable linkKeywords
Female reproductive system diseases
Hypoxic environment
Non-coding RNAs
Hypoxia-inducible factor-1 (HIF-1)
用户登录
还没有账号?
立即注册