Advances in DNA damage induced by environmental chemical carcinogens
Review Article
Genome Instability & Disease , 3 317–330 (2022)
Abstract
Chemical carcinogens, which are exogenous DNA-damaging agents, are ubiquitous in both the industry and living environments. The carcinogens pose a threat to the integrity of genetic information by causing diverse types of DNA damage through various pathways. Subsequently, the organism activates a DNA damage response mechanism to repair the damage caused by chemical carcinogens. However, incorrect or ineffective repair processes, along with DNA damage accumulation, may lead to genomic instability and mutations, which play a critical role in carcinogenesis. However, the types of DNA damage induced by a large variety of chemical carcinogens may vary due to the different physicochemical properties of chemicals and ultimately affect the carcinogenic process. In this review, we discuss the mechanisms of DNA damage and ultimately carcinogenesis by various environmental chemical carcinogens, namely heavy metals, polycyclic aromatic hydrocarbons, and aromatic amines. We also summarize the potential types of DNA damage that are closely associated with carcinogens.
Introduction
Cancer control has always been a challenge in the medical field. In addition to genetic factors, numerous environmental factors, including chemical, physical, and biological carcinogens, contribute to cancer occurrence and development (Belpomme et al., 2007). Approximately 80% of human cancers are caused by exposure to chemical carcinogens (Higginson, 1993). More than sixty chemicals, which are widespread in the industry and living environments, have been identified as Group 1 carcinogens by the International Agency for Research on Cancer (IARC) (Ian et al., 2022).
Most chemical carcinogens are exogenous DNA-damaging agents, which threaten the integrity of the genetic information. To counter this threat, through billions of years of evolution, living organisms have developed DNA damage response (DDR) systems, which detect and repair DNA damage (Jackson & Bartek, 2009). However, a faulty repair mechanism can cause the accumulation of DNA errors, which are a major source of genomic instability and mutations (Guo et al., 2019; Saitoh & Oda, 2021; Schiewer & Knudsen, 2019), leading to the inactivation of tumor suppressor genes and activation of oncogenes. Thus, DNA damage is regarded as an early event in carcinogenesis (Basu, 2018; Roos et al., 2016). Identifying chemical-induced DNA damage and exploring its role in carcinogenesis may contribute to cancer prevention and therapy. However, due to the diversity in chemicals, the types of DNA damage induced by different physicochemical properties of chemical carcinogens may vary. In this review, we focus on major common chemical carcinogens and describe their role in inducing DNA damage and ultimately carcinogenesis, according to chemical classifications.
Types of DNA damage
Exposure to carcinogens causes various types of DNA damage, such as single-stand breaks (SSBs), double-strand breaks (DSBs), bulky DNA adducts, DNA base modification, and intra/inter-strand crosslinks (Barnes et al., 2018; Poirier, 2012) (Fig. 1).
Fig. 1Types of DNA damage
DNA single-strand break
DNA SSBs are discontinuities in one strand of the DNA double helix and are usually associated with damage or mismatches at the 5'- and/or 3'-terminus. These are the most common type of DNA damage in cells, occurring several times per cell per day. DNA SSBs can arise from oxidized nucleotides/bases during oxidative stress, intermediate products of DNA repair pathways, and aborted activities of cellular enzymes. If DNA SSBs are not repaired quickly or accurately, they will subsequently develop into DNA DSBs (Higo et al. 2017), which pose a serious threat to genetic stability and cell survival (Abbotts & Wilson, 2017).
DNA double-strand breaks
DSBs occur when two complementary DNA strands are simultaneously broken at the same location or in close proximity to each other. Although only 10–50 DNA DSBs occur per cell per day, which is 1/20–1/30 of DNA SSBs, DSBs are highly deleterious, because they can lead to cell death, as well as deletions, translocations, and DNA fusions, if unrepaired or improperly repaired. DSBs are mainly caused by ionizing radiation and environmental pollutant exposures. In addition, DSBs can be caused directly or indirectly by other types of DNA damage. For example, replication forks progress to SSBs or inter-strand crosslinks, which might result in further DNA double-strand damage during cell replication. Accumulation of DSB damage leads to genetic instability, which in turn may increase the risk of cancer development (Khanna & Jackson, 2001).
Bulky DNA adducts
Bulky DNA adducts are indicators of genotoxic aromatic chemical exposure and reflect an individual's capacity to activate carcinogens metabolically and repair DNA damage. Most carcinogens require metabolic activation, and bulky DNA adducts result from the covalent interactions of electrophilic chemical carcinogens with the nucleophilic sites in DNA (Ewa & Danuta, 2017). DNA adducts of these carcinogens can be formed on various ring nitrogen, exocyclic nitrogen, and oxygen atoms of nucleobases. The type of DNA adducts formed depends on the structure of the reactive chemical, nature of the electrophile, and ability of the compound to intercalate with DNA (Hwa Yun et al., 2020), which may direct specific nucleophilic sites on DNA bases to form adducts. DNA adducts interfere with DNA transcription and replication and, if not repaired, can lead to cancer-specific mutations. Therefore, the formation of DNA adducts is a critical step in initiating carcinogenesis.
DNA base modification
DNA base modification is induced by oxidation, methylation, and alkylation changes (Gavande et al., 2016; Lord & Ashworth, 2012). Exposure to certain chemicals can directly or indirectly generate reactive oxygen species (ROS), which can lead to oxidative stress (Klaunig, 2018). The nitrogenous bases of DNA are easily oxidized by endogenous and exogenous oxidants, causing extensive oxidative DNA damage (Marnett, 2000). In addition to chain scission due to a direct attack on the phosphate backbone, nitrogenous bases can be modified by free radicals. If these lesions are not repaired or repair attempts fail, mutations and strand breaks subsequently occur, permanently altering the DNA sequence (Klages-Mundt & Li, 2017). Thus, DNA oxidation products are direct risk factors for genome stability. Guanine is most easily destroyed by oxidants owing to its low reduction potential. It generally pairs with cytosine, whereas the most common type of oxidized base damage, 8-oxo-7,8-dihydroguanine (8-oxoG), may lead to its mismatches with adenine through conformational changes (Poetsch, 2020). This is a common pathway for oxidative DNA damage-induced mutations. Therefore, 8-oxoG is the most abundant form of oxidative DNA damage, which has been extensively studied. Alkylation is the most mutagenic type of base lesions, mainly from alkylating agents’ exposure, such as formaldehyde, ethanol, and polycyclic aromatic hydrocarbons (Bauer et al., 2015). O6-alkylG is the most likely alkylation site to cause mutations (Drabløs et al., 2004). Adding alkyl groups at the guanine O6 site causes G:C → T:A transversion. Transferring the methyl of S-adenosylmethionine to the base causes DNA methylation. The methylation of cytosine to 5-methylcytosine at CpG site is a typical event of DNA base modification. Abnormal DNA methylation patterns at the CpG sites are often observed in cancer (Li et al., 2013).
Inter-/intra-strand crosslinks
Unwinding the DNA double helix is essential for cellular processes, such as DNA replication and transcription. Cross-linking reagents function by forming irreversible covalent crosslinks between two nucleotide residues of the same DNA strand (i.e., intra-strand crosslinks) or opposite strands (i.e., inter-strand crosslinks), hindering DNA strand separation. Thus, DNA cross-linking is extremely toxic to cells and forms the molecular basis for cellular chromosomal aberrations that affect cellular function and DNA replication.
Carcinogenic chemical-induced DNA damage
Carcinogenic chemicals are extremely diverse in structure and function and can be divided into the following two categories: direct-acting and indirect-acting carcinogens, according to the difference in inducing DNA damage directly or indirectly, respectively (Lutz, 1986). Direct-acting carcinogens, due to their electrophilic groups, can directly induce DNA damage by interacting with nitrogen and oxygen atoms in DNA and other cellular components without the need for metabolic activation or any molecular modification (Cohen & Arnold, 2011; Ravanat & Douki, 2016). Due to the relative inactivity of the parent compound, indirect-acting carcinogens typically require metabolic bioactivation by intracellular cytochrome P450 enzymes to carcinogenic metabolites or reactive intermediates, such as polycyclic aromatic hydrocarbons, heterocyclic aromatic amines, and N-nitrosamines, which can exert genotoxic effects (Harris et al., 2020). Most chemical carcinogens belong to this category.
Heavy metals
Almost all heavy metals are toxic (Khanam et al., 2020; Shahjahan et al., 2022). However, owing to their chemical and physiological properties, heavy metals are still widely used in industrial fields, such as alloying, smelting, and manufacturing commercial products (Ali et al., 2019). In addition to industrial production, waste from industrial processes is a major source of environmental metal pollution and human metal exposure (Vardhan et al., 2019). Heavy metals such as arsenic, cadmium (Cd), and chromium are classified as group 1 carcinogens by the IARC (Ian et al., 2022). Numerous epidemiological studies have shown that occupational exposure to these heavy metals is associated with increased risks of several severe cancers (Khlifi et al., 2013).
Several common mechanisms are involved in the development of cancers induced by heavy metals. Heavy metals cause redox processes that convert high-valent metal ions to low-valent metal ions while also generating a lot of free radicals, which trigger oxidative stress responses and cause various types of DNA damage. Additionally, heavy metals can inhibit the DNA repair pathways by interacting with DNA repair proteins or binding directly to the zinc finger structural domain, leading to the unrepaired or improperly repaired DNA damages (Hartwig, 1998; Hartwig & Schwerdtle, 2002).
Cadmium
The heavy metal Cd is an environmental contaminant found in natural and industrial emissions (Lin et al., 2018; Schwartz & Reis, 2000). Cd is highly toxic and exposure to it causes lung and kidney cancers (García-Esquinas et al., 2014; Il'yasova & Schwartz, 2005). The effects of Cd on genome stability are presumably indirect, mainly via an increase in oxidative stress, causing DNA damage, and inhibiting DNA damage repair (Joseph, 2009; Rani et al., 2014). Oxidative stress can occur indirectly by forming complexes with small peptides and proteins through sulfhydryl groups (SH) (Bishak et al., 2015). It can also be generated by replacing redox-active bio-metals (e.g., iron and copper) in the cytoplasm and membrane proteins through the Fenton reaction (Filipic et al., 2006), leading to various types of DNA damage, including oxidative DNA damage, DNA strand breaks, and DNA–protein crosslinks (Fatur et al., 2002; Tarhonska et al., 2022). Inhibition of DNA repair is also an important mechanism in Cd-induced cancers, resulting in insufficient repair mechanisms and accumulation of DNA damages, thus promoting cancer development (Giaginis et al., 2006). Malignant cells transformed in vitro by exposure to low doses of Cd present abnormal gene expression profiles in DNA repair signaling pathways (Oldani et al., 2020; Tan et al., 2019), with DNA SSBs and DSBs, which induce micronuclei, gene mutations, and genomic instability (Filipič, 2012). Cd can interact with DNA repair proteins to inhibit major DNA repair pathways. For example, Cd can inhibite the base excision repair by reducing the activity of 8-oxoguanine glycosylase (OGG1) (Bravard et al., 2010) and downregulate the binding of XPA protein to DNA impeding nucleotide excision repair (Kopera et al., 2004), or interference with mismatch recognition proteins, MSH2-MSH6 and MSH2-MSH3, to initiate DNA mismatch repair (Banerjee & Flores-Rozas, 2005; Wieland et al., 2009). In addition, Cd can alter the structure and function of p53 by displacing zinc, thus impairing p53 activity (Hamann et al., 2012; Hartwig, 2010; Yu et al., 2011).
Arsenic
Arsenic exposure mainly originates from arsenic-contaminated water or organisms growing in arsenic-contaminated soil (Mondal et al., 2021). The most common toxic inorganic forms are arsenic (III) and arsenic (V) (Yamamura & Amachi, 2014). Arsenic (III) is transported into cells via aquaporin 9, a water/glycerol transporting protein (Stamatelos et al., 2011); arsenic (V) enters cells through phosphate transporters (Chen et al., 2021). Purine nucleoside phosphorylase reduces arsenic (V) to the more toxic arsenic (III) (Németi & Gregus, 2007). Long-term arsenic exposure is associated with increased risks of various cancers in the bladder, lung, kidney, liver, prostate, and skin (Li et al., 2022a; Maiti et al., 2012; Mostafa et al., 2008; Saint-Jacques et al., 2014; Wang et al., 2014). Arsenic does not directly interact with DNA but leads to cancer mainly by inducing oxidative stress and inhibiting DNA repair pathways (Chen et al., 2019; Huang et al., 2004; Nail et al., 2022). Oxidative stress can generate ROS and reactive nitrogen species through mitochondrial dysfunction, antioxidant imbalance, and the activation of NADPH oxidase and nitric oxide synthase. These species attack DNA bases, cause DNA damage, and generate DNA oxidative damage products such as 8-hydroxy-2′-deoxy-guanosine, 5-methylcytosine, and 5-hydroxymethylcytosine, which are widely used as biomarkers for DNA oxidative damage (De Vizcaya-Ruiz et al., 2009; Niedzwiecki et al., 2015; Tsai et al., 2021; Wang et al., 2021). Chronic arsenic exposure can induce malignant transformation of various cells, including human bronchial epithelial cells (Stueckle et al., 2012), rat liver epithelial cells (Liu & Waalkes, 2008), human prostate epithelial RWPE-1 cells (Treas et al., 2013), and mouse embryonic fibroblasts (Barguilla et al., 2020), causing oxidative DNA damage (Tokar et al., 2014). In addition to its independent toxicity, arsenic mainly acts as an auxiliary carcinogen, enhancing the carcinogenic effects of other carcinogens at environmental concentrations (Burns et al., 2004; Li et al., 2019; Zhou et al., 2019, 2021). Thus, the DNA repair systems are highly sensitive to arsenic exposure even at low concentrations (Zhou et al., 2021). The primary mechanism for co-carcinogenesis is the inhibition of DNA repair pathways by direct binding to zinc finger domains, which can alter the activity of DNA repair proteins involved in the repair of nucleotide excision, base excision, DSB, and mismatch (Zhou et al., 2021). Chronic arsenic exposure is more likely to lead to faulty repairs which destabilize the genome, ultimately causing cancer (Nail et al., 2022). Several epigenetic changes have also been observed in the arsenic-induced DNA damage. For example, the DNA methylation biomarker N7-methylguanine is elevated after arsenic exposure (Tsai et al., 2021), and the histone demethylase JHDM2A inhibits nucleosides in arsenic-induced lesions acid levels express DDB2, which is a key factor in DNA repair (Li et al., 2022a).
Chromium (VI)
Chromium is abundant in the earth’s crust and is often used in industrial production. It can coexist in multiple valence states in the environment; however, only chromium (III) and (VI) are considerably toxic (Sawicka et al., 2021) and are important carcinogens of the digestive and respiratory systems (Balali-Mood et al., 2021; Deng et al., 2019; Yatera et al., 2018). Chromium (VI) is more carcinogenic than the other valence states mainly because it is absorbed by the cell and rapidly reduced to intermediates chromium (V), chromium (IV), and chromium (III), and producing a large amount of active hydroxyl radicals (Luo et al., 1996). These species can induce various types of DNA damage, including DNA adduct formation, SSBs, DSBs, and the crosslinks of DNA duplexes and DNA–protein (Arakawa et al., 2012; DeLoughery et al., 2015; Ferreira et al., 2019; VonHandorf et al., 2021; Wang et al., 2017; Wise et al., 2008). Epidemiological studies have shown significant positive associations between environmental exposure to chromium and DNA damage levels and the risk of lung, stomach, and oral cancer (Sharma et al., 2012; Welling et al., 2015; Yuan et al., 2011). Long-term exposure to chromium (VI) can cause DNA DSBs (DeLoughery et al., 2015) and reduce E2F1 transcription factor levels. E2F1 is the predominant transcription factor for the DNA repair protein RAD51, which is involved in DNA DSB repair via homologous recombination (Choi & Kim, 2019). Chromium (VI) can inhibit the formation of RAD51 nuclear foci, leading to the failure to repair DSBs (Browning & Wise, 2017b; Browning et al., 2016b; Qin et al., 2014; Speer et al., 2021). In addition, chromium (VI) specifically targets the genomic regions around the binding sites for the transcription factors CTCF and AP1 in the nucleosomal architecture of euchromatin. These changes alter the DNA-binding capacity of key transcription factors, which in turn leads to altered chromatin states that disrupt gene transcription in functionally relevant biological processes (VonHandorf et al., 2018). This leads to the main tumor manifestations, such as malignant cell migration and invasion (Ma et al., 2022).
Polycyclic aromatic hydrocarbons
Polycyclic aromatic hydrocarbons are ubiquitous persistent environmental pollutants formed mainly from the incomplete combustion of organic matter. Several polycyclic aromatic hydrocarbons have been identified in the environment, some of which are carcinogens, such as benzo[a]pyrene (Bukowska et al., 2022; Reizer et al., 2019). Benzo[a]pyrene is also an indicator of carcinogenicity of other polycyclic aromatic hydrocarbons (Agency for Toxic Substances & Disease Registry, 2022). Deng et al. treated male mice with benzo[a]pyrene for 24 h and observed DNA damage in the liver and lung, which was significantly higher than that in the kidney, stomach, and brain (Deng et al., 2018), thus identifying the target organs for benzo[a]pyrene. Numerous epidemiological studies have associated benzo[a]pyrene exposure with increased risks for many types of cancers, including those of the lung, kidney, liver, bladder, breast, skin, and stomach (Amadou et al., 2021; Dybing et al., 2008; Enuneku et al., 2021; Gürbüz et al., 2021; Labib et al., 2012; Nakayama et al., 1984; Xue et al., 2022; Zhao et al., 2018). Although benzo[a]pyrene has been classified as a human carcinogen, it is an indirect carcinogen. Its metabolic activation is catalyzed by cytochrome P450 (CYP) enzymes (e.g., CYP1A1 and CYP1B1) to produce the ultimate carcinogenic form of benzo(a)pyrene, 7,8-dihydrodiol-9,10-epoxide (BPDE) (Moorthy et al., 2015; Reed et al., 2018; Tsutomu & Yoshiaki, 2003). As a reactive electrophile, BPDE can be alkylated and bind covalently to the exocyclic amino group of guanine and nucleophilic sites in DNA, forming N2-guanine adducts BPDE-N2-dG, which can induce a frameshift or base substitution mutation, such as G to T transversion, as well as GC → TA and GC → CG mutations (Zhao et al., 2006). Therefore, BPDE has the potential to activate oncogenes and cause cancers by DNA damage accumulation, increasing the frequency of DNA base mutations (Shimada, 2006; Tung et al., 2010; Wei et al., 1991).
Generally, DNA damage activates a cell cycle checkpoint, leading to the induction of transcriptional programs and the enhancement of DNA repair pathways (Sancar et al., 2004). DNA damage caused by benzo[a]pyrene exposure mainly involves BPDE-DNA adducts, base mismatches, and DNA DSBs. Correspondingly, nucleotide excision repair mechanisms eliminate DNA adducts, and base excision repair maintains genome integrity and corrects modifications in the DNA bases (Allmann et al., 2020b; Kress et al., 2019), whereas homologous recombination is involved in the repair of DNA DSBs (Li & Heyer, 2008). These DNA repair mechanisms are inhibited after benzo[a]pyrene exposure (Allmann et al., 2020b; Tung et al., 2014; Yang et al., 2007), leading to aberrant DNA damage repair, which is key to the accumulation of irreparable DNA damage (Tung et al., 2014).
Aromatic amines
Aromatic amines (AAs) are a class of chemicals found in consumer products such as dyes, pesticides, and tobacco smoke. One in eight of all known or suspected human carcinogens exists as or can be converted to an AA (Wang et al., 2019). Several AAs have been identified as carcinogens and mutagens, including o-toluidine (o-Tol), 2-aminonaphthalene, and 4-aminobiphenyl (4-ABP), which are the most important risk factors for bladder cancer (Bellamri et al., 2019; Nauwelaers et al., 2011; Tajima et al., 2020).
The key to bladder cancer caused by these AAs is the formation of DNA adducts (Bellamri et al., 2019; Tajima et al., 2020). For example, approximately 80% of 4-ABP molecules form DNA adducts at the C8 atoms of guanine (Nauwelaërs et al., 2013), synthesized as described for N-(deoxyguanosin-8-yl) adducts. The remaining 4-ABP molecules are activated to carcinogenic N-oxidation intermediates by cytochrome P450 1A2 to produce the genotoxic N-hydroxy-4-ABP metabolite, which can further interact with DNA to form adducts, causing oxidative DNA damage and DNA DSBs (Marnett, 2000; Shahab et al., 2013; Torino et al., 2001). In addition, 4-ABP can cause bladder cancer by inducing mutations characterized by a high frequency of G:C → T:A transversion, and appropriate alkyl substitution can modify the mutagenicity of 4-ABP (Chen et al., 2005; Glende et al., 2001; Yoon et al., 2012).
DNA damage repair in chemical carcinogenesis
In response to different types of DNA damage induced by endogenous or exogenous stimuli, organisms mount DDR to sense, eliminate, or tolerate damage to maintain genetic stability and integrity (Chang et al., 2017; Sadoughi et al., 2021). DNA damage accumulation and aberrant DNA damage repair play a critical role in the accumulation of mutations. Unrepaired or incorrectly repaired DNA damage may ultimately lead to genomic instability and mutations, which increase the risks of cancers. DNA repair pathways are parts of the DDR, including base excision repair, nucleotide excision repair, mismatch repair, non-homologous end joining (NHEJ), and homologous recombination (HR). In general, specific types of DNA damages are amenable to being repaired by particular DNA repair pathways. For example, exposure to PM2.5, Cr(VI), and benzo[a]pyrene can easily lead to DSBs and are commonly accompanied with the reduced expression of DNA repair proteins (e.g., Lig4, Dclre1c, and RAD51), indicating the inhibition of HR and NHEJ (Allmann et al., 2020; Browning & Wise, 2017a; Browning et al., 2016a; Zeng et al., 2021). Both HR and NHEJ are two common ways for repairing DSBs. Compared to HR, NHEJ is faster but also more error-prone, because it disregards sequence homology, does not rely on homologous DNA sequences, and only rejoins damaged DNA ends via DNA ligases (Ciccia & Elledge, 2010); therefore, NHEJ in non-homologous DNA sequences makes aberrant repair of DSBs a potential mechanism for mutagenesis. Therefore, targeting DNA repair pathways is a promising therapeutic strategy for cancers (Huang & Zhou, 2021; Jin & Oh, 2019; Motegi et al., 2019). The DNA damage repair and carcinogenic mechanisms of the chemical carcinogens investigated in this study are shown in Table 1. A better understanding of the complex interaction between DNA damage and repair is critical for elucidating chemical carcinogenesis mechanisms and studying the etiology of cancer in the future.
Table 1 DNA damage repair and carcinogenic mechanism of chemical carcinogens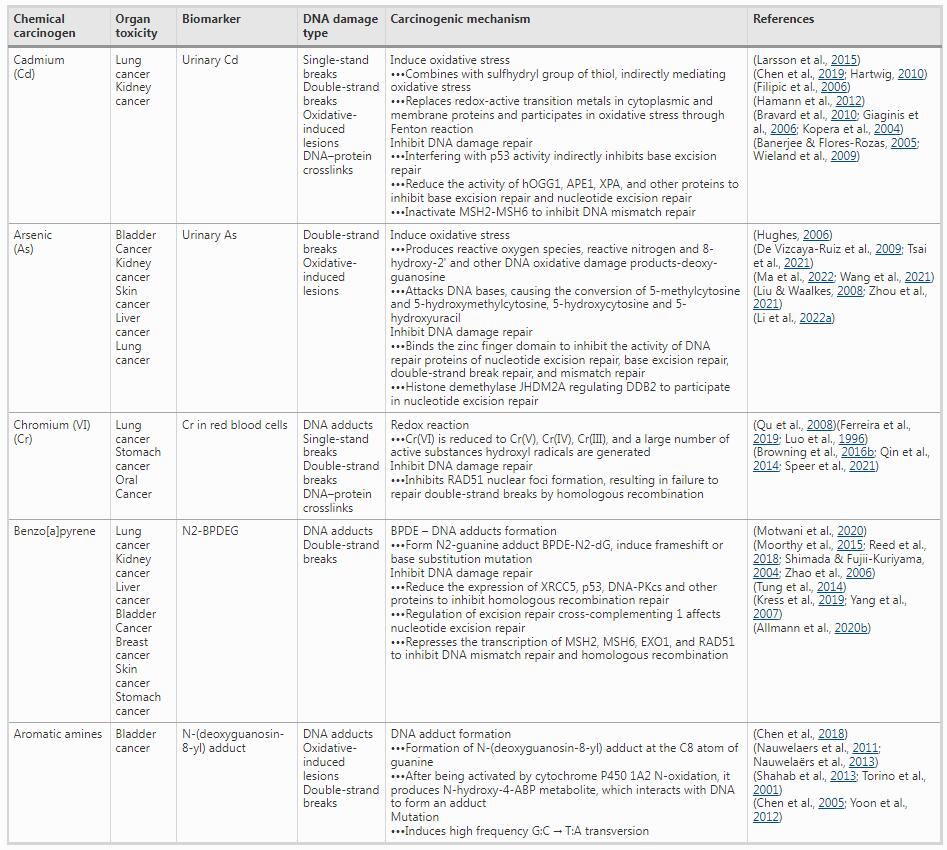
OGG1 8-oxoguanine glycosylase, APE1 apurinic-apyrimidinic endonuclease I, XPA xeroderma pigmentosum group A, MSH2 mutS homolog 2, MSH6 mutS homolog 6, JHDM2A lysine demethylase 3A, DDB2 damage specific DNA binding protein 2, RAD51 RADiation sensitive 51, BPDE benzo(a)pyrene, 7,8-dihydrodiol-9,10-epoxide, XRCC5 X-ray repair cross complementing 5, DNA-PKcs DNA-dependent protein kinase catalytic subunit, EXO1 exonuclease 1
Epigenetic regulation in DNA damage carcinogenesis
There is a consensus on the regulatory function of epigenetics in the occurrence and development of cancers. DNA damage induced by exposure to chemical carcinogens is one of the crucial mechanisms in chemical carcinogenesis. Epigenetic alterations such as silencing of histone markers or histone demethylation, DNA hypermethylation, and abnormal expressions of noncoding RNAs can co-regulate DNA damage that is induced by chemical carcinogens through influencing the transcription of key transcription factors and the expression of DNA repair genes, eventually leading to the accumulation of DNA damage. Methylation is the function that has received the most attention among the roles of epigenetic changes by exposure to diverse environmental carcinogens (Verghese et al., 2022). For example, carcinogenic heavy metals inhibit the production of DNA repair genes by DNA hypermethylation, histone marker silencing, or histone demethylation, leading to enhanced genetic damage (Hu et al., 2018; Li et al., 2021, 2022b; Ren et al., 2019; Wang et al., 2018). Furthermore, benzo(a)pyrene and 4-ABP exposure causes CpG methylation, which improves the binding of BPDE adducts and N–OH-4-ABP to the CpG site and leads to p53 gene mutation in cancer (Feng et al., 2002; Wang et al., 2013). An active area of research in recent years has been the regulatory role of abnormal expressions of noncoding RNAs in environmental chemical carcinogenesis. For example, circRNAs, such as circ_Cabin1 and circNIPBL, can downregulate the functional DNA damage repair molecules Lig4, Dclre1c, and PARP1, inhibiting the NHEJ and base excision repair pathways and promoting DNA damage after 4-(methylnitrosamino)-1-(3-pyridyl)-1-butanone and PM2.5 exposure, respectively (Liu et al., 2022; Zeng et al., 2021). LncRNA MT1DP might bind to SMARCAL1, competitively inhibit latter's interaction with RPA complex, and stimulate DNA replication through the ATR pathway to regulate Cd-induced DNA damage (Feng et al., 2022). However, the role of epigenetics in DNA damage-initiated carcinogenesis remains largely unknown and requires further exploration.
Conclusions and future perspectives
Common chemical carcinogens mainly form DNA adducts through the metabolic activation of CYP450 enzymes or generate ROS and hydroxyl radicals, which cause DNA damage and have an indirect carcinogenic effect. The following need to be considered: (1) almost all environmental chemical carcinogens can cause DNA damage; however, no specific type of DNA damage is unique to all chemical carcinogens. (2) Exposure to one chemical carcinogen can simultaneously cause multiple types of DNA damage. (3) The types of DNA damage differ with the chemical carcinogen. Chemical type-based DNA damage mechanisms help expand our understanding of the toxic effects of novel chemicals. It should also be noted that when DNA damage is induced by environmental chemical carcinogens, the activation or inhibition of various DNA repair pathways influences the development of cancer. The involvement of epigenetics in DNA damage repair is essential in environmental carcinogen exposure. For the purpose of elucidating the mechanisms of chemical carcinogenesis and investigating the etiology of cancer, it is crucial to have a deeper understanding of the intricate relationships between DNA damage and epigenetics.
References
Abbotts, R., & Wilson, D. M., 3rd. (2017). Coordination of DNA single strand break repair. Free Radical Biology & Medicine, 107, 228–244. https://doi.org/10.1016/j.freeradbiomed.2016.11.039
Agency for Toxic Substances and Disease Registry. (2022, April 14). Guidance for calculating benzo(a)pyrene equivalents for cancer evaluations of polycyclic aromatic hydrocarbons. Atlanta, GA: U.S. Department of Health and Human Services, Public Health Service. Retrieved August 22, 2022 from https://www.atsdr.cdc.gov/pha-guidance/resources/ATSDR-PAH-Guidance-508.pdf
Ali, H., Khan, E., & Ilahi, I. (2019). Environmental chemistry and ecotoxicology of hazardous heavy metals: Environmental persistence, toxicity, and bioaccumulation. Journal of Chemistry, 2019(1), 1–14. https://doi.org/10.1155/2019/6730305
Allmann, S., Mayer, L., Olma, J., Kaina, B., Hofmann, T. G., Tomicic, M. T., & Christmann, M. (2020). Benzo[a]pyrene represses DNA repair through altered E2F1/E2F4 function marking an early event in DNA damage-induced cellular senescence. Nucleic Acids Research, 48(21), 12085–12101. https://doi.org/10.1093/nar/gkaa965
Amadou, A., Praud, D., Coudon, T., Deygas, F., Grassot, L., Faure, E., Couvidat, F., Caudeville, J., Bessagnet, B., Salizzoni, P., Gulliver, J., Leffondré, K., Severi, G., Mancini, F. R., & Fervers, B. (2021). Risk of breast cancer associated with long-term exposure to benzo[a]pyrene (BaP) air pollution: Evidence from the French E3N cohort study. Environment International, 149, 106399. https://doi.org/10.1016/j.envint.2021.106399
Arakawa, H., Weng, M. W., Chen, W. C., & Tang, M. S. (2012). Chromium (VI) induces both bulky DNA adducts and oxidative DNA damage at adenines and guanines in the p53 gene of human lung cells. Carcinogenesis, 33(10), 1993–2000. https://doi.org/10.1093/carcin/bgs237
Balali-Mood, M., Naseri, K., Tahergorabi, Z., Khazdair, M. R., & Sadeghi, M. (2021). Toxic mechanisms of five heavy metals: Mercury, lead, chromium, cadmium, and arsenic. Frontiers in Pharmacology, 12, 643972. https://doi.org/10.3389/fphar.2021.643972
Banerjee, S., & Flores-Rozas, H. (2005). Cadmium inhibits mismatch repair by blocking the ATPase activity of the MSH2-MSH6 complex. Nucleic Acids Research, 33(4), 1410–1419. https://doi.org/10.1093/nar/gki291
Barguilla, I., Peremartí, J., Bach, J., Marcos, R., & Hernández, A. (2020). Role of As3mt and Mth1 in the genotoxic and carcinogenic effects induced by long-term exposures to arsenic in MEF cells. Toxicology and Applied Pharmacology, 409, 115303. https://doi.org/10.1016/j.taap.2020.115303
Barnes, J. L., Zubair, M., John, K., Poirier, M. C., & Martin, F. L. (2018). Carcinogens and DNA damage. Chemical Society Transactions, 46(5), 1213–1224. https://doi.org/10.1042/bst20180519
Basu, A. K. (2018). DNA damage, mutagenesis and cancer. International Journal of Molecular Sciences, 19(4), 970. https://doi.org/10.3390/ijms19040970
Bauer, N. C., Corbett, A. H., & Doetsch, P. W. (2015). The current state of eukaryotic DNA base damage and repair. Nucleic Acids Research, 43(21), 10083–10101. https://doi.org/10.1093/nar/gkv1136
Bellamri, M., Yao, L., Bonala, R., Johnson, F., Von Weymarn, L. B., & Turesky, R. J. (2019). Bioactivation of the tobacco carcinogens 4-aminobiphenyl (4-ABP) and 2-amino-9H-pyrido[2,3-b]indole (AαC) in human bladder RT4 cells. Archives of Toxicology, 93(7), 1893–1902. https://doi.org/10.1007/s00204-019-02486-7
Belpomme, D., Irigaray, P., Sasco, A. J., Newby, J. A., Howard, V., Clapp, R., & Hardell, L. (2007). The growing incidence of cancer: Role of lifestyle and screening detection (Review). International Journal of Oncology, 30(5), 1037–1049. https://doi.org/10.3892/ijo.30.5.1037
Bishak, Y. K., Payahoo, L., Osatdrahimi, A., & Nourazarian, A. (2015). Mechanisms of cadmium carcinogenicity in the gastrointestinal tract. Asian Pacific Journal of Cancer Prevention, 16(1), 9–21. https://doi.org/10.7314/apjcp.2015.16.1.9
Bravard, A., Campalans, A., Vacher, M., Gouget, B., Levalois, C., Chevillard, S., & Radicella, J. P. (2010). Inactivation by oxidation and recruitment into stress granules of hOGG1 but not APE1 in human cells exposed to sub-lethal concentrations of cadmium. Mutation Research, 685(1–2), 61–69. https://doi.org/10.1016/j.mrfmmm.2009.09.013
Browning, C. L., & Wise, J. P., Sr. (2017a). Prolonged exposure to particulate chromate inhibits RAD51 nuclear import mediator proteins. Toxicology and Applied Pharmacology, 331, 101–107. https://doi.org/10.1016/j.taap.2017.05.030
Browning, C. L., Qin, Q., Kelly, D. F., Prakash, R., Vanoli, F., Jasin, M., & Wise, J. P., Sr. (2016). Prolonged particulate hexavalent chromium exposure suppresses homologous recombination repair in human lung cells. Toxicological Sciences, 153(1), 70–78. https://doi.org/10.1093/toxsci/kfw103
Bukowska, B., Mokra, K., & Michalowicz, J. (2022). Benzo[a]pyrene-environmental occurrence, human exposure, and mechanisms of toxicity. International Journal of Molecular Sciences, 23(11), 6348. https://doi.org/10.3390/ijms23116348
Burns, F. J., Uddin, A. N., Wu, F., Nádas, A., & Rossman, T. G. (2004). Arsenic-induced enhancement of ultraviolet radiation carcinogenesis in mouse skin: A dose-response study. Environmental Health Perspectives, 112(5), 599–603. https://doi.org/10.1289/ehp.6655
Chang, H. H. Y., Pannunzio, N. R., Adachi, N., & Lieber, M. R. (2017). Non-homologous DNA end joining and alternative pathways to double-strand break repair. Nature Reviews Molecular Cell Biology, 18(8), 495–506. https://doi.org/10.1038/nrm.2017.48
Chen, T., Mittelstaedt, R. A., Beland, F. A., Heflich, R. H., Moore, M. M., & Parsons, B. L. (2005). 4-Aminobiphenyl induces liver DNA adducts in both neonatal and adult mice but induces liver mutations only in neonatal mice. International Journal of Cancer, 117(2), 182–187. https://doi.org/10.1002/ijc.21173
Chen, Z., Zhang, Y., & Vouros, P. (2018). Recent technical and biological development in the analysis of biomarker N-deoxyguanosine-C8-4-aminobiphenyl. Journal of Chromatography. b, Analytical Technologies in the Biomedical and Life Sciences, 1087–1088, 49–60. https://doi.org/10.1016/j.jchromb.2018.04.041
Chen, Q. Y., DesMarais, T., & Costa, M. (2019). Metals and Mechanisms of Carcinogenesis. Annual Review of Pharmacology and Toxicology, 59, 537–554. https://doi.org/10.1146/annurev-pharmtox-010818-021031
Chen, J., Nadar, V. S., & Rosen, B. P. (2021). Aquaglyceroporin AqpS from Sinorhizobium meliloti conducts both trivalent and pentavalent methylarsenicals. Chemosphere, 270, 129379. https://doi.org/10.1016/j.chemosphere.2020.129379
Choi, E. H., & Kim, K. P. (2019). E2F1 facilitates DNA break repair by localizing to break sites and enhancing the expression of homologous recombination factors. Experimental and Molecular Medicine, 51(9), 1–12. https://doi.org/10.1038/s12276-019-0307-2
Ciccia, A., & Elledge, S. J. (2010). The DNA damage response: Making it safe to play with knives. Molecular Cell, 40(2), 179–204. https://doi.org/10.1016/j.molcel.2010.09.019
Cohen, S. M., & Arnold, L. L. (2011). Chemical carcinogenesis. Toxicological Sciences, 120(Suppl 1), S76-92. https://doi.org/10.1093/toxsci/kfq365
De Vizcaya-Ruiz, A., Barbier, O., Ruiz-Ramos, R., & Cebrian, M. E. (2009). Biomarkers of oxidative stress and damage in human populations exposed to arsenic. Mutation Research, 674(1–2), 85–92. https://doi.org/10.1016/j.mrgentox.2008.09.020
DeLoughery, Z., Luczak, M. W., Ortega-Atienza, S., & Zhitkovich, A. (2015). DNA double-strand breaks by Cr(VI) are targeted to euchromatin and cause ATR-dependent phosphorylation of histone H2AX and its ubiquitination. Toxicological Sciences, 143(1), 54–63. https://doi.org/10.1093/toxsci/kfu207
Deng, C., Dang, F., Gao, J., Zhao, H., Qi, S., & Gao, M. (2018). Acute benzo[a]pyrene treatment causes different antioxidant response and DNA damage in liver, lung, brain, stomach and kidney. Heliyon, 4(11), e00898. https://doi.org/10.1016/j.heliyon.2018.e00898
Deng, Y., Wang, M., Tian, T., Lin, S., Xu, P., Zhou, L., Dai, C., Hao, Q., Wu, Y., Zhai, Z., Zhu, Y., Zhuang, G., & Dai, Z. (2019). The Effect of hexavalent chromium on the incidence and mortality of human cancers: A meta-analysis based on published epidemiological cohort studies. Frontiers in Oncology, 9, 24. https://doi.org/10.3389/fonc.2019.00024
Drabløs, F., Feyzi, E., Aas, P. A., Vaagbø, C. B., Kavli, B., Bratlie, M. S., Peña-Diaz, J., Otterlei, M., Slupphaug, G., & Krokan, H. E. (2004). Alkylation damage in DNA and RNA–repair mechanisms and medical significance. DNA Repair (amst), 3(11), 1389–1407. https://doi.org/10.1016/j.dnarep.2004.05.004
Dybing, E., O’Brien, J., Renwick, A. G., & Sanner, T. (2008). Risk assessment of dietary exposures to compounds that are genotoxic and carcinogenic–an overview. Toxicology Letters, 180(2), 110–117. https://doi.org/10.1016/j.toxlet.2008.05.007
Enuneku, A., Ogbeide, O., Okpara, B., Kubeyinje, B. F., Job, O., Asemota, C. O., Imoobe, T., & Ezemonye, L. I. (2021). Ingestion and dermal cancer risk via exposure to polycyclic aromatic hydrocarbon-contaminated soils in an oil-producing community, Niger Delta. Nigeria. Environmental Toxicology and Chemistry, 40(1), 261–271. https://doi.org/10.1002/etc.4906
Ewa, B., & Danuta, M. (2017). Polycyclic aromatic hydrocarbons and PAH-related DNA adducts. Journal of Applied Genetics, 58(3), 321–330. https://doi.org/10.1007/s13353-016-0380-3
Fatur, T., Tusek, M., Falnoga, I., Scancar, J., Lah, T. T., & Filipic, M. (2002). DNA damage and metallothionein synthesis in human hepatoma cells (HepG2) exposed to cadmium. Food and Chemical Toxicology, 40(8), 1069–1076. https://doi.org/10.1016/s0278-6915(02)00058-3
Feng, Z., Hu, W., Rom, W. N., Beland, F. A., & Tang, M. S. (2002). N-hydroxy-4-aminobiphenyl-DNA binding in human p53 gene: Sequence preference and the effect of C5 cytosine methylation. Biochemistry, 41(20), 6414–6421. https://doi.org/10.1021/bi020093s
Feng, W., Qi, Z., Dong, Z., Liu, W., Xu, M., Gao, M., & Liu, S. (2022). LncRNA MT1DP promotes cadmium-induced DNA replication stress by inhibiting chromatin recruitment of SMARCAL1. Science of the Total Environment, 807(Pt 3), 151078. https://doi.org/10.1016/j.scitotenv.2021.151078
Ferreira, L. M. R., Cunha-Oliveira, T., Sobral, M. C., Abreu, P. L., Alpoim, M. C., & Urbano, A. M. (2019). Impact of carcinogenic chromium on the cellular response to proteotoxic stress. International Journal of Molecular Sciences, 20(19), 4901. https://doi.org/10.3390/ijms20194901
Filipič, M. (2012). Mechanisms of cadmium induced genomic instability. Mutation Research, 733(1–2), 69–77. https://doi.org/10.1016/j.mrfmmm.2011.09.002
Filipic, M., Fatur, T., & Vudrag, M. (2006). Molecular mechanisms of cadmium induced mutagenicity. Human and Experimental Toxicology, 25(2), 67–77. https://doi.org/10.1191/0960327106ht590oa
García-Esquinas, E., Pollan, M., Tellez-Plaza, M., Francesconi, K. A., Goessler, W., Guallar, E., Umans, J. G., Yeh, J., Best, L. G., & Navas-Acien, A. (2014). Cadmium exposure and cancer mortality in a prospective cohort: The strong heart study. Environmental Health Perspectives, 122(4), 363–370. https://doi.org/10.1289/ehp.1306587
Gavande, N. S., VanderVere-Carozza, P. S., Hinshaw, H. D., Jalal, S. I., Sears, C. R., Pawelczak, K. S., & Turchi, J. J. (2016). DNA repair targeted therapy: The past or future of cancer treatment? Pharmacology & Therapeutics, 160, 65–83. https://doi.org/10.1016/j.pharmthera.2016.02.003
Giaginis, C., Gatzidou, E., & Theocharis, S. (2006). DNA repair systems as targets of cadmium toxicity. Toxicology and Applied Pharmacology, 213(3), 282–290. https://doi.org/10.1016/j.taap.2006.03.008
Glende, C., Schmitt, H., Erdinger, L., Engelhardt, G., & Boche, G. (2001). Transformation of mutagenic aromatic amines into non-mutagenic species by alkyl substituents. Part I. Alkylation ortho to the amino function. Mutation Research/Genetic Toxicology and Environmental Mutagenesis, 498(1–2), 19–37. https://doi.org/10.1016/s1383-5718(01)00259-5
Guo, H., Liu, H., Wu, H., Cui, H., Fang, J., Zuo, Z., Deng, J., Li, Y., Wang, X., & Zhao, L. (2019). Nickel carcinogenesis mechanism: DNA damage. International Journal of Molecular Sciences, 20(19), 4690. https://doi.org/10.3390/ijms20194690
Gürbüz, B., Topal, C. S., Sobay, R., Alkurt, G., & Zemheri, I. E. (2021). Molecular and immunohistochemical evaluation of BAP-1 antibody in bladder cancer and comparison with luminal-basal subtyping. Pathology, Research and Practice, 217, 153308. https://doi.org/10.1016/j.prp.2020.153308
Hamann, I., König, C., Richter, C., Jahnke, G., & Hartwig, A. (2012). Impact of cadmium on hOGG1 and APE1 as a function of the cellular p53 status. Mutation Research, 736(1–2), 56–63. https://doi.org/10.1016/j.mrfmmm.2011.05.006
Harris, J. B., Hartman, J. H., Luz, A. L., Wilson, J. Y., Dinyari, A., & Meyer, J. N. (2020). Zebrafish CYP1A expression in transgenic Caenorhabditis elegans protects from exposures to benzo[a]pyrene and a complex polycyclic aromatic hydrocarbon mixture. Toxicology, 440, 152473. https://doi.org/10.1016/j.tox.2020.152473
Hartwig, A. (1998). Carcinogenicity of metal compounds: Possible role of DNA repair inhibition. Toxicology Letters, 102–103, 235–239. https://doi.org/10.1016/s0378-4274(98)00312-9
Hartwig, A. (2010). Mechanisms in cadmium-induced carcinogenicity: Recent insights. BioMetals, 23(5), 951–960. https://doi.org/10.1007/s10534-010-9330-4
Hartwig, A., & Schwerdtle, T. (2002). Interactions by carcinogenic metal compounds with DNA repair processes: Toxicological implications. Toxicology Letters, 127(1–3), 47–54. https://doi.org/10.1016/s0378-4274(01)00482-9
Higginson, J. (1993). Environmental carcinogenesis. Cancer, 72(3 Suppl), 971–977. https://doi.org/10.1002/1097-0142(19930801)72:3+%3c971::aid-cncr2820721304%3e3.0.co;2-g
Higo, T., Naito, A. T., Sumida, T., Shibamoto, M., Okada, K., Nomura, S., Nakagawa, A., Yamaguchi, T., Sakai, T., Hashimoto, A., Kuramoto, Y., Ito, M., Hikoso, S., Akazawa, H., Lee, J. K., Shiojima, I., McKinnon, P. J., Sakata, Y., & Komuro, I. (2017). DNA single-strand break-induced DNA damage response causes heart failure. Nature Communications, 8, 15104. https://doi.org/10.1038/ncomms15104
Hu, G., Li, P., Cui, X., Li, Y., Zhang, J., Zhai, X., Yu, S., Tang, S., Zhao, Z., Wang, J., & Jia, G. (2018). Cr(VI)-induced methylation and down-regulation of DNA repair genes and its association with markers of genetic damage in workers and 16HBE cells. Environmental Pollution, 238, 833–843. https://doi.org/10.1016/j.envpol.2018.03.046
Huang, R., & Zhou, P. K. (2021). DNA damage repair: Historical perspectives, mechanistic pathways and clinical translation for targeted cancer therapy. Signal Transduction and Targeted Therapy, 6(1), 254. https://doi.org/10.1038/s41392-021-00648-7
Huang, C., Ke, Q., Costa, M., & Shi, X. (2004). Molecular mechanisms of arsenic carcinogenesis. Molecular and Cellular Biochemistry, 255(1–2), 57–66. https://doi.org/10.1023/b:mcbi.0000007261.04684.78
Hughes, M. F. (2006). Biomarkers of exposure: A case study with inorganic arsenic. Environmental Health Perspectives, 114(11), 1790–1796. https://doi.org/10.1289/ehp.9058
Hwa Yun, B., Guo, J., Bellamri, M., & Turesky, R. J. (2020). DNA adducts: Formation, biological effects, and new biospecimens for mass spectrometric measurements in humans. Mass Spectrometry Reviews, 39(1–2), 55–82. https://doi.org/10.1002/mas.21570
Ian, C., Mary, S.-B., Lamia, B.-T., Nathan, D., Aline, d. C., Fatiha, E. G., Yann, G., Federica, M., David, R., Leslie, S., Eero, S., Roland, W., Heidi, M., Jennifer, N., Niree, K., Solène, Q., & Sandrine, R. (2022, July 1). IARC monographs on the identification of carcinogenic hazards to humans. World Health Organization: International Agency for Research on Cancer. Retrieved August 22, 2022 from https://monographs.iarc.who.int/agents-classified-by-the-iarc/
Il’yasova, D., & Schwartz, G. G. (2005). Cadmium and renal cancer. Toxicology and Applied Pharmacology, 207(2), 179–186. https://doi.org/10.1016/j.taap.2004.12.005
Jackson, S. P., & Bartek, J. (2009). The DNA-damage response in human biology and disease. Nature, 461(7267), 1071–1078. https://doi.org/10.1038/nature08467
Jin, M. H., & Oh, D. Y. (2019). ATM in DNA repair in cancer. Pharmacology and Therapeutics, 203, 107391. https://doi.org/10.1016/j.pharmthera.2019.07.002
Joseph, P. (2009). Mechanisms of cadmium carcinogenesis. Toxicology and Applied Pharmacology, 238(3), 272–279. https://doi.org/10.1016/j.taap.2009.01.011
Khanam, R., Kumar, A., Nayak, A. K., Shahid, M., Tripathi, R., Vijayakumar, S., Bhaduri, D., Kumar, U., Mohanty, S., Panneerselvam, P., Chatterjee, D., Satapathy, B. S., & Pathak, H. (2020). Metal(loid)s (As, Hg, Se, Pb and Cd) in paddy soil: Bioavailability and potential risk to human health. Science of the Total Environment, 699, 134330. https://doi.org/10.1016/j.scitotenv.2019.134330
Khanna, K. K., & Jackson, S. P. (2001). DNA double-strand breaks: Signaling, repair and the cancer connection. Nature Genetics, 27(3), 247–254. https://doi.org/10.1038/85798
Khlifi, R., Olmedo, P., Gil, F., Hammami, B., Chakroun, A., Rebai, A., & Hamza-Chaffai, A. (2013). Arsenic, cadmium, chromium and nickel in cancerous and healthy tissues from patients with head and neck cancer. Science of the Total Environment, 452–453, 58–67. https://doi.org/10.1016/j.scitotenv.2013.02.050
Klages-Mundt, N. L., & Li, L. (2017). Formation and repair of DNA-protein crosslink damage. Science China-Life Sciences, 60(10), 1065–1076. https://doi.org/10.1007/s11427-017-9183-4
Klaunig, J. E. (2018). Oxidative stress and cancer. Current Pharmaceutical Design, 24(40), 4771–4778. https://doi.org/10.2174/1381612825666190215121712
Kopera, E., Schwerdtle, T., Hartwig, A., & Bal, W. (2004). Co(II) and Cd(II) substitute for Zn(II) in the zinc finger derived from the DNA repair protein XPA, demonstrating a variety of potential mechanisms of toxicity. Chemical Research in Toxicology, 17(11), 1452–1458. https://doi.org/10.1021/tx049842s
Kress, J. M., Dio, L. D., Heck, L., Pulliero, A., Izzotti, A., Laarmann, K., Fritz, G., & Kaina, B. (2019). Human primary endothelial cells are impaired in nucleotide excision repair and sensitive to benzo[a]pyrene compared with smooth muscle cells and pericytes. Scientific Reports, 9(1), 13800. https://doi.org/10.1038/s41598-019-49953-w
Labib, S., Yauk, C., Williams, A., Arlt, V. M., Phillips, D. H., White, P. A., & Halappanavar, S. (2012). Subchronic oral exposure to benzo(a)pyrene leads to distinct transcriptomic changes in the lungs that are related to carcinogenesis. Toxicological Sciences, 129(1), 213–224. https://doi.org/10.1093/toxsci/kfs177
Larsson, S. C., Orsini, N., & Wolk, A. (2015). Urinary cadmium concentration and risk of breast cancer: A systematic review and dose-response meta-analysis. American Journal of Epidemiology, 182(5), 375–380. https://doi.org/10.1093/aje/kwv085
Li, X., & Heyer, W. D. (2008). Homologous recombination in DNA repair and DNA damage tolerance. Cell Research, 18(1), 99–113. https://doi.org/10.1038/cr.2008.1
Li, J., Braganza, A., & Sobol, R. W. (2013). Base excision repair facilitates a functional relationship between Guanine oxidation and histone demethylation. Antioxidants & Redox Signaling, 18(18), 2429–2443. https://doi.org/10.1089/ars.2012.5107
Li, Y., He, M., Chen, B., & Hu, B. (2019). Inhibition of arsenite methylation induces synergistic genotoxicity of arsenite and benzo(a)pyrene diol epoxide in SCC-7 cells. Metallomics, 11(1), 176–182. https://doi.org/10.1039/c8mt00217g
Li, P., Zhang, X., Murphy, A. J., Costa, M., Zhao, X., & Sun, H. (2021). Downregulation of hedgehog-interacting protein (HHIP) contributes to hexavalent chromium-induced malignant transformation of human bronchial epithelial cells. Carcinogenesis, 42(1), 136–147. https://doi.org/10.1093/carcin/bgaa085
Li, C., Zhang, A., Hu, T., Yang, Y., Tang, S., & Li, J. (2022a). Histone demethylase JHDM2A participates in the repair of arsenic-induced DNA damage in L-02 cells by regulating DDB2. Toxicology and Industrial Health. https://doi.org/10.1177/07482337221098319
Li, C., Zhang, A., Hu, T., Yang, Y., Tang, S., & Li, J. (2022b). Histone demethylase JHDM2A participates in the repair of arsenic-induced DNA damage in L-02 cells by regulating DDB2. Toxicology and Industrial Health, 38(6), 365–376. https://doi.org/10.1177/07482337221098319
Lin, X., Peng, L., Xu, X., Chen, Y., Zhang, Y., & Huo, X. (2018). Connecting gastrointestinal cancer risk to cadmium and lead exposure in the Chaoshan population of Southeast China. Environmental Science and Pollution Reseach, 25(18), 17611–17619. https://doi.org/10.1007/s11356-018-1914-5
Liu, J., & Waalkes, M. P. (2008). Liver is a target of arsenic carcinogenesis. Toxicological Sciences, 105(1), 24–32. https://doi.org/10.1093/toxsci/kfn120
Liu, Y., Hua, Q., Li, M., Li, X., Chen, W., Zeng, H., Diao, Q., Shi, C., Ling, Y., & Jiang, Y. (2022). Circular RNA circNIPBL promotes NNK-induced DNA damage in bronchial epithelial cells via the base excision repair pathway. Archives of Toxicology, 96(7), 2049–2065. https://doi.org/10.1007/s00204-022-03297-z
Lord, C. J., & Ashworth, A. (2012). The DNA damage response and cancer therapy. Nature, 481(7381), 287–294. https://doi.org/10.1038/nature10760
Luo, H., Lu, Y., Mao, Y., Shi, X., & Dalal, N. S. (1996). Role of chromium(IV) in the chromium(VI)-related free radical formation, dG hydroxylation, and DNA damage. Journal of Inorganic Biochemistry, 64(1), 25–35. https://doi.org/10.1016/0162-0134(95)00241-3
Lutz, W. K. (1986). Quantitative evaluation of DNA binding data for risk estimation and for classification of direct and indirect carcinogens. Journal of Cancer Research and Clinical Oncology, 112(2), 85–91. https://doi.org/10.1007/bf00404387
Ma, Y., Li, S., Ye, S., Hu, D., Wei, L., & Xiao, F. (2022). Hexavalent chromium triggers hepatocytes premature senescence via the GATA4/NF-κB signaling pathway mediated by the DNA damage response. Ecotoxicology and Environmental Safety, 239, 113645. https://doi.org/10.1016/j.ecoenv.2022.113645
Maiti, S., Chattopadhyay, S., Deb, B., Samanta, T., Maji, G., Pan, B., Ghosh, A., & Ghosh, D. (2012). Antioxidant and metabolic impairment result in DNA damage in arsenic-exposed individuals with severe dermatological manifestations in Eastern India. Environmental Toxicology, 27(6), 342–350. https://doi.org/10.1002/tox.20647
Marnett, L. J. (2000). Oxyradicals and DNA damage. Carcinogenesis, 21(3), 361–370. https://doi.org/10.1093/carcin/21.3.361
Mondal, D., Rahman, M. M., Suman, S., Sharma, P., Siddique, A. B., Rahman, M. A., Bari, A., Kumar, R., Bose, N., Singh, S. K., Ghosh, A., & Polya, D. A. (2021). Arsenic exposure from food exceeds that from drinking water in endemic area of Bihar, India. Science of the Total Environment, 754, 142082. https://doi.org/10.1016/j.scitotenv.2020.142082
Moorthy, B., Chu, C., & Carlin, D. J. (2015). Polycyclic aromatic hydrocarbons: From metabolism to lung cancer. Toxicological Sciences, 145(1), 5–15. https://doi.org/10.1093/toxsci/kfv040
Mostafa, M. G., McDonald, J. C., & Cherry, N. M. (2008). Lung cancer and exposure to arsenic in rural Bangladesh. Occupational and Environmental Medicine, 65(11), 765–768. https://doi.org/10.1136/oem.2007.037895
Motegi, A., Masutani, M., Yoshioka, K. I., & Bessho, T. (2019). Aberrations in DNA repair pathways in cancer and therapeutic significances. Seminars in Cancer Biology, 58, 29–46. https://doi.org/10.1016/j.semcancer.2019.02.005
Motwani, H. V., Westberg, E., Lindh, C., Abramsson-Zetterberg, L., & Törnqvist, M. (2020). Serum albumin adducts, DNA adducts and micronuclei frequency measured in benzo[a]pyrene-exposed mice for estimation of genotoxic potency. Mutation Research, Genetic Toxicology and Environmental Mutagenesis, 849, 503127. https://doi.org/10.1016/j.mrgentox.2019.503127
Nail, A. N., McCaffrey, L. M., Banerjee, M., Ferragut Cardoso, A. P., & States, J. C. (2022). Chronic arsenic exposure suppresses ATM pathway activation in human keratinocytes. Toxicology and Applied Pharmacology, 446, 116042. https://doi.org/10.1016/j.taap.2022.116042
Nakayama, J., Yuspa, S. H., & Poirier, M. C. (1984). Benzo(a)pyrene-DNA adduct formation and removal in mouse epidermis in vivo and in vitro: Relationship of DNA binding to initiation of skin carcinogenesis. Cancer Research, 44(9), 4087–4095.
Nauwelaers, G., Bessette, E. E., Gu, D., Tang, Y., Rageul, J., Fessard, V., Yuan, J. M., Yu, M. C., Langouët, S., & Turesky, R. J. (2011). DNA adduct formation of 4-aminobiphenyl and heterocyclic aromatic amines in human hepatocytes. Chemical Research in Toxicology, 24(6), 913–925. https://doi.org/10.1021/tx200091y
Nauwelaërs, G., Bellamri, M., Fessard, V., Turesky, R. J., & Langouët, S. (2013). DNA adducts of the tobacco carcinogens 2-amino-9H-pyrido[2,3-b]indole and 4-aminobiphenyl are formed at environmental exposure levels and persist in human hepatocytes. Chemical Research in Toxicology, 26(9), 1367–1377. https://doi.org/10.1021/tx4002226
Németi, B., & Gregus, Z. (2007). Glutathione-dependent reduction of arsenate by glycogen phosphorylase a reaction coupled to glycogenolysis. Toxicological Sciences, 100(1), 36–43. https://doi.org/10.1093/toxsci/kfm211
Niedzwiecki, M. M., Liu, X., Hall, M. N., Thomas, T., Slavkovich, V., Ilievski, V., Levy, D., Alam, S., Siddique, A. B., Parvez, F., Graziano, J. H., & Gamble, M. V. (2015). Sex-specific associations of arsenic exposure with global DNA methylation and hydroxymethylation in leukocytes: Results from two studies in Bangladesh. Cancer Epidemiology Biomarkers and Prevention, 24(11), 1748–1757. https://doi.org/10.1158/1055-9965.Epi-15-0432
Oldani, M., Manzoni, M., Villa, A. M., Stefanini, F. M., Melchioretto, P., Monti, E., Forcella, M., Urani, C., & Fusi, P. (2020). Cadmium elicits alterations in mitochondrial morphology and functionality in C3H10T1/2Cl8 mouse embryonic fibroblasts. Biochimica Et Biophysica Acta-General Subjects, 1864(8), 129568. https://doi.org/10.1016/j.bbagen.2020.129568
Poetsch, A. R. (2020). The genomics of oxidative DNA damage, repair, and resulting mutagenesis. Computational and Structural Biotechnology Journal, 18, 207–219. https://doi.org/10.1016/j.csbj.2019.12.013
Poirier, M. C. (2012). Chemical-induced DNA damage and human cancer risk. Discovery Medicine, 14(77), 283–288.
Qin, Q., Xie, H., Wise, S. S., Browning, C. L., Thompson, K. N., Holmes, A. L., & Wise, J. P., Sr. (2014). Homologous recombination repair signaling in chemical carcinogenesis: Prolonged particulate hexavalent chromium exposure suppresses the Rad51 response in human lung cells. Toxicological Sciences, 142(1), 117–125. https://doi.org/10.1093/toxsci/kfu175
Qu, Q., Li, X., An, F., Jia, G., Liu, L., Watanabe-Meserve, H., Koenig, K., Cohen, B., Costa, M., Roy, N., Zhong, M., Chen, L. C., Liu, S., & Yan, L. (2008). CrVI exposure and biomarkers: Cr in erythrocytes in relation to exposure and polymorphisms of genes encoding anion transport proteins. Biomarkers, 13(5), 467–477. https://doi.org/10.1080/13547500802099574
Rani, A., Kumar, A., Lal, A., & Pant, M. (2014). Cellular mechanisms of cadmium-induced toxicity: A review. International Journal of Environmental Health Research, 24(4), 378–399. https://doi.org/10.1080/09603123.2013.835032
Ravanat, J.-L., & Douki, T. (2016). UV and ionizing radiations induced DNA damage, differences and similarities. Radiation Physics and Chemistry, 128, 92–102. https://doi.org/10.1016/j.radphyschem.2016.07.007
Reed, L., Mrizova, I., Barta, F., Indra, R., Moserova, M., Kopka, K., Schmeiser, H. H., Wolf, C. R., Henderson, C. J., Stiborova, M., Phillips, D. H., & Arlt, V. M. (2018). Cytochrome b 5 impacts on cytochrome P450-mediated metabolism of benzo[a]pyrene and its DNA adduct formation: Studies in hepatic cytochrome b 5 /P450 reductase null (HBRN) mice. Archives of Toxicology, 92(4), 1625–1638. https://doi.org/10.1007/s00204-018-2162-7
Reizer, E., Csizmadia, I. G., Palotas, A. B., Viskolcz, B., & Fiser, B. (2019). Formation mechanism of benzo(a)pyrene: one of the most carcinogenic polycyclic aromatic hydrocarbons (PAH). Molecules, 24(6), 1040. https://doi.org/10.3390/molecules24061040
Ren, X., Xia, B., Chen, Z., Chen, X., Wu, D., Lu, W., Luo, N., Zhou, L., Liu, W., Yang, X., & Liu, J. (2019). Short-term and long-term exposure to hexavalent chromium alters 53BP1 via H3K18ac and H3K27ac. Chemosphere, 229, 284–294. https://doi.org/10.1016/j.chemosphere.2019.04.113
Roos, W. P., Thomas, A. D., & Kaina, B. (2016). DNA damage and the balance between survival and death in cancer biology. Nature Reviews Cancer, 16(1), 20–33. https://doi.org/10.1038/nrc.2015.2
Sadoughi, F., Maleki Dana, P., Asemi, Z., & Yousefi, B. (2021). DNA damage response and repair in osteosarcoma: Defects, regulation and therapeutic implications. DNA Repair, 102, 103105. https://doi.org/10.1016/j.dnarep.2021.103105
Saint-Jacques, N., Parker, L., Brown, P., & Dummer, T. J. (2014). Arsenic in drinking water and urinary tract cancers: A systematic review of 30 years of epidemiological evidence. Environmental Health, 13, 44. https://doi.org/10.1186/1476-069x-13-44
Saitoh, T., & Oda, T. (2021). DNA damage response in multiple myeloma: the role of the tumor microenvironment. Cancers (Basel), 13(3), 504. https://doi.org/10.3390/cancers13030504
Sancar, A., Lindsey-Boltz, L. A., Unsal-Kacmaz, K., & Linn, S. (2004). Molecular mechanisms of mammalian DNA repair and the DNA damage checkpoints. Annual Review of Biochemistry, 73, 39–85. https://doi.org/10.1146/annurev.biochem.73.011303.073723
Sawicka, E., Jurkowska, K., & Piwowar, A. (2021). Chromium (III) and chromium (VI) as important players in the induction of genotoxicity - current view. Annals of Agricultural and Environmental Medicine, 28(1), 1–10. https://doi.org/10.26444/aaem/118228
Schiewer, M. J., & Knudsen, K. E. (2019). DNA damage response in prostate cancer. Cold Spring Harbor Perspectives in Medicine. https://doi.org/10.1101/cshperspect.a030486
Schwartz, G. G., & Reis, I. M. (2000). Is cadmium a cause of human pancreatic cancer? Cancer Epidemiology Biomarkers and Prevention, 9(2), 139–145.
Shahab, U., Moinuddin Ahmad, S., Dixit, K., Habib, S., Alam, K., & Ali, A. (2013). Genotoxic effect of N-hydroxy-4-acetylaminobiphenyl on human DNA: Implications in bladder cancer. PLoS One, 8(1), e53205. https://doi.org/10.1371/journal.pone.0053205
Shahjahan, M., Taslima, K., Rahman, M. S., Al-Emran, M., Alam, S. I., & Faggio, C. (2022). Effects of heavy metals on fish physiology - a review. Chemosphere, 300, 134519. https://doi.org/10.1016/j.chemosphere.2022.134519
Sharma, P., Bihari, V., Agarwal, S. K., Verma, V., Kesavachandran, C. N., Pangtey, B. S., Mathur, N., Singh, K. P., Srivastava, M., & Goel, S. K. (2012). Groundwater contaminated with hexavalent chromium [Cr (VI)]: A health survey and clinical examination of community inhabitants (Kanpur, India). PLoS One, 7(10), e47877. https://doi.org/10.1371/journal.pone.0047877
Shimada, T. (2006). Xenobiotic-metabolizing enzymes involved in activation and detoxification of carcinogenic polycyclic aromatic hydrocarbons. Drug Metabolism and Pharmacokinetics, 21(4), 257–276. https://doi.org/10.2133/dmpk.21.257
Shimada, T., & Fujii-Kuriyama, Y. (2004). Metabolic activation of polycyclic aromatic hydrocarbons to carcinogens by cytochromes P450 1A1 and 1B1. Cancer Science, 95(1), 1–6. https://doi.org/10.1111/j.1349-7006.2004.tb03162.x
Speer, R. M., Toyoda, J. H., Croom-Perez, T. J., Liu, K. J., & Wise, J. P. (2021). Particulate hexavalent chromium inhibits E2F1 leading to reduced RAD51 nuclear foci formation in human lung cells. Toxicological Sciences, 181(1), 35–46. https://doi.org/10.1093/toxsci/kfab019
Stamatelos, S. K., Brinkerhoff, C. J., Isukapalli, S. S., & Georgopoulos, P. G. (2011). Mathematical model of uptake and metabolism of arsenic(III) in human hepatocytes - incorporation of cellular antioxidant response and threshold-dependent behavior. BMC System Biology, 5, 16. https://doi.org/10.1186/1752-0509-5-16
Stueckle, T. A., Lu, Y., Davis, M. E., Wang, L., Jiang, B. H., Holaskova, I., Schafer, R., Barnett, J. B., & Rojanasakul, Y. (2012). Chronic occupational exposure to arsenic induces carcinogenic gene signaling networks and neoplastic transformation in human lung epithelial cells. Toxicology and Applied Pharmacology, 261(2), 204–216. https://doi.org/10.1016/j.taap.2012.04.003
Tajima, Y., Toyoda, T., Hirayama, Y., Matsushita, K., Yamada, T., Ogawa, K., Watanabe, K., Takamura-Enya, T., Totsuka, Y., Wakabayashi, K., & Miyoshi, N. (2020). Novel o-toluidine metabolite in rat urine associated with urinary bladder carcinogenesis. Chemical Research in Toxicology, 33(7), 1907–1914. https://doi.org/10.1021/acs.chemrestox.0c00098
Tan, H. W., Liang, Z. L., Yao, Y., Wu, D. D., Mo, H. Y., Gu, J., Chiu, J. F., Xu, Y. M., & Lau, A. T. Y. (2019). Lasting DNA damage and aberrant DNA repair gene expression profile are associated with post-chronic cadmium exposure in human bronchial epithelial cells. Cells. https://doi.org/10.3390/cells8080842
Tarhonska, K., Lesicka, M., Janasik, B., Roszak, J., Reszka, E., Braun, M., Kołacińska-Wow, A., & Jabłońska, E. (2022). Cadmium and breast cancer - current state and research gaps in the underlying mechanisms. Toxicology Letters, 361, 29–42. https://doi.org/10.1016/j.toxlet.2022.03.003
Tokar, E. J., Kojima, C., & Waalkes, M. P. (2014). Methylarsonous acid causes oxidative DNA damage in cells independent of the ability to biomethylate inorganic arsenic. Archives of Toxicology, 88(2), 249–261. https://doi.org/10.1007/s00204-013-1141-2
Torino, J. L., Burger, M. S., Reznikoff, C. A., & Swaminathan, S. (2001). Role of TP53 in repair of N-(deoxyguanosin-8-yl)-4-aminobiphenyl adducts in human transitional cell carcinoma of the urinary bladder. Carcinogenesis, 22(1), 147–154. https://doi.org/10.1093/carcin/22.1.147
Treas, J., Tyagi, T., & Singh, K. P. (2013). Chronic exposure to arsenic, estrogen, and their combination causes increased growth and transformation in human prostate epithelial cells potentially by hypermethylation-mediated silencing of MLH1. Prostate, 73(15), 1660–1672. https://doi.org/10.1002/pros.22701
Tsai, T. L., Kuo, C. C., Hsu, L. I., Tsai, S. F., Chiou, H. Y., Chen, C. J., Hsu, K. H., & Wang, S. L. (2021). Association between arsenic exposure, DNA damage, and urological cancers incidence: A long-term follow-up study of residents in an arseniasis endemic area of northeastern Taiwan. Chemosphere, 266, 129094. https://doi.org/10.1016/j.chemosphere.2020.129094
Tsutomu, S., & Yoshiaki, F.-K. (2003). Metabolic activation of polycyclic aromatic hydrocarbons to carcinogens by cytochromes P450 1A1 and 1B1. Cancer Science, 95(1), 1–6. https://doi.org/10.1111/j.1349-7006.2004.tb03162.x
Tung, J. N., Wu, H. H., Chiang, C. C., Tsai, Y. Y., Chou, M. C., Lee, H., & Cheng, Y. W. (2010). An association between BPDE-like DNA adduct levels and CYP1A1 and GSTM1 polymorphisma in pterygium. Molecular Vision, 16, 623–629.
Tung, E. W., Philbrook, N. A., Belanger, C. L., Ansari, S., & Winn, L. M. (2014). Benzo[a]pyrene increases DNA double strand break repair in vitro and in vivo: A possible mechanism for benzo[a]pyrene-induced toxicity. Mutation Research/genetic Toxicology and Environmental Mutagenesis, 760, 64–69. https://doi.org/10.1016/j.mrgentox.2013.12.003
Vardhan, K. H., Kumar, P. S., & Panda, R. C. (2019). A review on heavy metal pollution, toxicity and remedial measures: current trends and future perspectives. Journal of Molecular Liquids, 290, 111197. https://doi.org/10.1016/j.molliq.2019.111197
Verghese, M., Wilkinson, E., & He, Y. Y. (2022). Role of RNA modifications in carcinogenesis and carcinogen damage response. Molecular Carcinogenesis. https://doi.org/10.1002/mc.23418
VonHandorf, A., Sánchez-Martín, F. J., Biesiada, J., Zhang, H., Zhang, X., Medvedovic, M., & Puga, A. (2018). Chromium disrupts chromatin organization and CTCF access to its cognate sites in promoters of differentially expressed genes. Epigenetics, 13(4), 363–375. https://doi.org/10.1080/15592294.2018.1454243
VonHandorf, A., Zablon, H. A., Biesiada, J., Zhang, X., Medvedovic, M., & Puga, A. (2021). Hexavalent chromium promotes differential binding of CTCF to its cognate sites in euchromatin. Epigenetics, 16(12), 1361–1376. https://doi.org/10.1080/15592294.2020.1864168
Wang, H. T., Weng, M. W., Chen, W. C., Yobin, M., Pan, J., Chung, F. L., Wu, X. R., Rom, W., & Tang, M. S. (2013). Effect of CpG methylation at different sequence context on acrolein- and BPDE-DNA binding and mutagenesis. Carcinogenesis, 34(1), 220–227. https://doi.org/10.1093/carcin/bgs323
Wang, W., Cheng, S., & Zhang, D. (2014). Association of inorganic arsenic exposure with liver cancer mortality: A meta-analysis. Environmental Research, 135, 120–125. https://doi.org/10.1016/j.envres.2014.08.034
Wang, Y., Su, H., Gu, Y., Song, X., & Zhao, J. (2017). Carcinogenicity of chromium and chemoprevention: A brief update. OncoTargets and Therapy, 10, 4065–4079. https://doi.org/10.2147/ott.S139262
Wang, Z., Wu, J., Humphries, B., Kondo, K., Jiang, Y., Shi, X., & Yang, C. (2018). Upregulation of histone-lysine methyltransferases plays a causal role in hexavalent chromium-induced cancer stem cell-like property and cell transformation. Toxicology and Applied Pharmacology, 342, 22–30. https://doi.org/10.1016/j.taap.2018.01.022
Wang, S., Hanna, D., Sugamori, K. S., & Grant, D. M. (2019). Primary aromatic amines and cancer: Novel mechanistic insights using 4-aminobiphenyl as a model carcinogen. Pharmacology and Therapeutics, 200, 179–189. https://doi.org/10.1016/j.pharmthera.2019.05.004
Wang, Q., Wang, W., & Zhang, A. (2021). TET-mediated DNA demethylation plays an important role in arsenic-induced HBE cells oxidative stress via regulating promoter methylation of OGG1 and GSTP1. Toxicology in Vitro, 72, 105075. https://doi.org/10.1016/j.tiv.2020.105075
Wei, S. J., Chang, R. L., Wong, C. Q., Bhachech, N., Cui, X. X., Hennig, E., Yagi, H., Sayer, J. M., Jerina, D. M., & Preston, B. D. (1991). Dose-dependent differences in the profile of mutations induced by an ultimate carcinogen from benzo[a]pyrene. Proceedings of the National Academy of Sciences of the United States of America, 88(24), 11227–11230. https://doi.org/10.1073/pnas.88.24.11227
Welling, R., Beaumont, J. J., Petersen, S. J., Alexeeff, G. V., & Steinmaus, C. (2015). Chromium VI and stomach cancer: A meta-analysis of the current epidemiological evidence. Occupational and Environmental Medicine, 72(2), 151–159. https://doi.org/10.1136/oemed-2014-102178
Wieland, M., Levin, M. K., Hingorani, K. S., Biro, F. N., & Hingorani, M. M. (2009). Mechanism of cadmium-mediated inhibition of Msh2-Msh6 function in DNA mismatch repair. Biochemistry, 48(40), 9492–9502. https://doi.org/10.1021/bi9001248
Wise, S. S., Holmes, A. L., & Wise, J. P., Sr. (2008). Hexavalent chromium-induced DNA damage and repair mechanisms. Reviews on Environmental Health, 23(1), 39–57. https://doi.org/10.1515/reveh.2008.23.1.39
Xue, Y., Wang, L., Zhang, Y., Zhao, Y., & Liu, Y. (2022). Air pollution: A culprit of lung cancer. Journal of Hazardous Materials, 434, 128937. https://doi.org/10.1016/j.jhazmat.2022.128937
Yamamura, S., & Amachi, S. (2014). Microbiology of inorganic arsenic: From metabolism to bioremediation. Journal of Bioscience and Bioengineering, 118(1), 1–9. https://doi.org/10.1016/j.jbiosc.2013.12.011
Yang, J., Liu, X., Niu, P., Zou, Y., Gong, Z., Yuan, J., & Wu, T. (2007). Dynamic changes of XPA, XPC, XPF, XPG and ERCC1 protein expression and their correlations with levels of DNA damage in human bronchial epithelia cells exposed to benzo[a]pyrene. Toxicology Letters, 174(1–3), 10–17. https://doi.org/10.1016/j.toxlet.2007.08.004
Yatera, K., Morimoto, Y., Ueno, S., Noguchi, S., Kawaguchi, T., Tanaka, F., Suzuki, H., & Higashi, T. (2018). Cancer risks of hexavalent chromium in the respiratory tract. Journal of UOEH, 40(2), 157–172. https://doi.org/10.7888/juoeh.40.157
Yoon, J. I., Kim, S. I., Tommasi, S., & Besaratinia, A. (2012). Organ specificity of the bladder carcinogen 4-aminobiphenyl in inducing DNA damage and mutation in mice. Cancer Prevention Research, 5(2), 299–308. https://doi.org/10.1158/1940-6207.Capr-11-0309
Yu, X., Sidhu, J. S., Hong, S., Robinson, J. F., Ponce, R. A., & Faustman, E. M. (2011). Cadmium induced p53-dependent activation of stress signaling, accumulation of ubiquitinated proteins, and apoptosis in mouse embryonic fibroblast cells. Toxicological Sciences, 120(2), 403–412. https://doi.org/10.1093/toxsci/kfr010
Yuan, T. H., Lian Ie, B., Tsai, K. Y., Chang, T. K., Chiang, C. T., Su, C. C., & Hwang, Y. H. (2011). Possible association between nickel and chromium and oral cancer: A case-control study in central Taiwan. Science of the Total Environment, 409(6), 1046–1052. https://doi.org/10.1016/j.scitotenv.2010.11.038
Zeng, H., Li, M., Hua, Q., Liu, Y., Shao, Y., Diao, Q., Ling, Y., Zhang, H., Qiu, M., Zhu, J., Li, X., Zhang, R., & Jiang, Y. (2021). Circular RNA circ_Cabin1 promotes DNA damage in multiple mouse organs via inhibition of non-homologous end-joining repair upon PM(25) exposure. Archives of Toxicology, 95(10), 3235–3251. https://doi.org/10.1007/s00204-021-03138-5
Zhao, B., Wang, J., Geacintov, N. E., & Wang, Z. (2006). Poleta, Polzeta and Rev1 together are required for G to T transversion mutations induced by the (+)- and (-)-trans-anti-BPDE-N2-dG DNA adducts in yeast cells. Nucleic Acids Research, 34(2), 417–425. https://doi.org/10.1093/nar/gkj446
Zhao, G., Wang, Z., Huang, Y., Ye, L., Yang, K., Huang, Q., Chen, X., Li, G., Chen, Y., Wang, J., & Zhou, Y. (2018). Effects of benzoapyrene on migration and invasion of lung cancer cells functioning by TNF-α. Journal of Cell Biochemistry, 119(8), 6492–6500. https://doi.org/10.1002/jcb.26683
Zhou, X., Ding, X., Shen, J., Yang, D., Hudson, L. G., & Liu, K. J. (2019). Peroxynitrite contributes to arsenic-induced PARP-1 inhibition through ROS/RNS generation. Toxicology and Applied Pharmacology, 378, 114602. https://doi.org/10.1016/j.taap.2019.114602
Zhou, X., Speer, R. M., Volk, L., Hudson, L. G., & Liu, K. J. (2021). Arsenic co-carcinogenesis: Inhibition of DNA repair and interaction with zinc finger proteins. Seminars in Cancer Biology, 76, 86–98. https://doi.org/10.1016/j.semcancer.2021.05.009
Funding
This study was supported by the National Natural Science Foundation of China (82173483 to Z.Y. and 82130095 to J.Y.)
Author information
Authors and Affiliations
State Key Laboratory of Respiratory Disease, The First Affiliated Hospital of Guangzhou Medical University, Guangzhou, 510120, China
Han Zhang, Yun Zhou & Yiguo Jiang
Institute for Chemical Carcinogenesis, Guangzhou Medical University, Guangzhou, 511436, China
Han Zhang, Wenfeng Lu, Yun Zhou & Yiguo Jiang
Corresponding authors
Correspondence to Yun Zhou or Yiguo Jiang.
Ethics declarations
Conflict of interest
The authors declared no conflict of interest.
Rights and permissions
Springer Nature or its licensor (e.g. a society or other partner) holds exclusive rights to this article under a publishing agreement with the author(s) or other rightsholder(s); author self-archiving of the accepted manuscript version of this article is solely governed by the terms of such publishing agreement and applicable law.
About this article
Cite this article
Zhang, H., Lu, W., Zhou, Y. et al. Advances in DNA damage induced by environmental chemical carcinogens. GENOME INSTAB. DIS. 3, 317–330 (2022). https://doi.org/10.1007/s42764-022-00092-z
Received23 August 2022
Revised08 November 2022
Accepted08 November 2022
Published18 November 2022
Issue DateDecember 2022
DOIhttps://doi.org/10.1007/s42764-022-00092-z
Share this article
Anyone you share the following link with will be able to read this content:
Get shareable linkKeywords
Carcinogens
DNA damage
Cancers
Environmental chemicals
用户登录
还没有账号?
立即注册