DYNLL1 transcript abundance decreases during oocyte and follicular growth and is associated with fertility in mice
Original Research Paper
Genome Instability & Disease (2022)
Abstract
DNA repair efficiency is an important determinant of oocyte quality and female fertility. DYNLL1 (Dynein light chain 1) plays a part in DNA double-strand break (DSB) repair choice by promoting error-prone non-homologous end joining (NHEJ) repair and in contrast by limiting accurate and error-free homologous recombination (HR) repair. Here, we showed that DYNLL1 transcript abundance decreases gradually during oocyte and follicular growth in mice. In parallel, DYNLL1 mRNA transcript levels decline in the course of oocyte meiotic maturation in mice. Furthermore, our in silico analysis showed that ovaries from high-fertility mice with increased ovulation have lower DYNLL1 transcript abundance compared with that of control mice. Similarly, we found that the induction of ovulation with human chorionic gonadotropin (hCG) decreases DYNLL1 mRNA transcript levels in cumulus oocyte complexes in mice. We hypothesize that this decrease in DYNLL1 transcript abundance during oocyte and follicular growth and in ovulation might lead to relatively higher error-free HR repair and lower error-prone NHEJ repair events in mice, resulting in increased genome stability. Increased genome stability in mice with lower DYNLL1 levels might also contribute to better fertility performance. This paper highlights the need for further in vivo and mechanistic studies to better understand the role of DYNLL1 down-regulation in the maintenance of oocyte reserve and in oocyte quality and fertility.
Introduction
The decline in ovarian reserve accelerates with age from birth to menopause, resulting in decreased reproductive performance (Faddy et al., 1992; Hansen et al., 2008; Younis, 2011). DNA repair efficiency is an important determinant of oocyte aging in women (Titus et al., 2013). DNA double-strand breaks (DSB) accumulate in primordial follicles with age, in parallel to decreases in the expression of the key DSB repair genes, such as BRCA1, MRE11, Rad51, and ATM, suggesting an age-associated loss of oocyte quality within the primordial follicles (Titus et al., 2013). Therefore, DSB repair is vital for the maintenance of oocyte reserve in women (Titus et al., 2013). A better understanding of DNA repair during oocyte and follicular growth may be useful in the development of novel treatment strategies to slow down the depletion of ovarian follicle reserve and to delay menopause to later ages, thus improving the reproductive performance of women.
The growth of the oocyte is accompanied by the growth of the follicle (Clarke, 2017). Non-growing prophase-arrested oocytes present within the primordial follicles are the immature precursors of all ovulated oocytes (Clarke, 2017). During reproductive life, these non-growing or dormant primordial follicles begin to proliferate mitotically. They are then recruited into the growing pool, and at this stage, the follicles are termed “primary follicles” (Clarke, 2017; Da Silva-Buttkus et al., 2008; Hirshfield, 1991; Lee & Chang, 2019; Wright et al., 2020). Following the formation of secondary follicle, small antral follicle and large antral follicle, respectively; at the final stage of oocyte development, meiotic maturation occurs. With this event, immature oocyte at the germinal vesicle (GV) stage develops into mature metaphase II (MII) oocyte (Adhikari & Liu, 2014; Clarke, 2017; Conti et al., 2012; Coticchio et al., 2015; Holt et al., 2013). This meiotic maturation is physiologically induced by the release of luteinizing hormone (LH), which also induces ovulation (Clarke, 2017). Fertilization then triggers the completion of the second meiotic division (Clarke, 2017).
DYNLL1 (Dynein light chain 1 protein; alias LC8, PIN) plays a part in DSB repair choice by promoting toxic/error-prone non-homologous end joining (NHEJ) repair and in contrast by limiting accurate and error-free homologous recombination (HR) repair (Becker et al., 2018; Berkel & Cacan, 2021; He et al., 2018; West et al., 2019). It inhibits DNA end resection in HR, but promotes 53BP1-dependent NHEJ; therefore, its increased cellular levels might be associated with increased genomic instability (Becker et al., 2018; He et al., 2018; West et al., 2019). We also found that DYNLL1 is involved in the progression, aggressiveness, and chemoresistance of ovarian cancer, further highlighting its role in gynecological health (Berkel & Cacan, 2020, 2021). However, how DYNLL1 mRNA transcript levels change during oocyte and follicular growth, and how its cellular levels are correlated with fertility considering its function in DNA repair and thus in oocyte quality is currently unknown.
In the present study, we aim to analyze changes in DYNLL1 transcript abundance during oocyte and follicular growth and in the course of oocyte meiotic maturation in mice. We also studied how treatment with human chorionic gonadotropin (hCG) effects DYNLL1 mRNA transcript levels in cumulus oocyte complexes. Finally, we asked if ovaries from high-fertility mice which exhibit an increase in ovulation number have different DYNLL1 transcript abundance compared with that of control mice which have normal ovulation number, to identify any possible correlation between DYNLL1 transcript abundance and fertility in mice.
Methods
Datasets
Following gene expression datasets from GEO (Gene Expression Omnibus, a public functional genomics data repository) were used in this study: GSE3351 (sample size (n) = 60) (Pan et al., 2005), GSE5668 (n = 18) (Salisbury et al., 2009; Su et al., 2007), GSE11667 (n = 48) (Pan et al., 2008), GSE4260 (n = 24) (Hernandez-Gonzalez et al., 2006), and GSE11113 (n = 72) (Vanselow et al., 2008). These datasets were selected to be analyzed in the current study, since they contain data for DYNLL1 mRNA transcript levels during oocyte and follicular growth, or they contain DYNLL1 transcript abundance data in terms of fertility or of treatment with human chorionic gonadotropin hormone. In GSE11113 dataset, high-fertility mouse line (FL1) represents the mouse line with higher mean number of corpora lutea at the first day of pregnancy, with higher number of ova flushed from the oviducts and with increased mean litter size and litter weight (Vanselow et al., 2008). Experiment summaries and overall design can be accessed using these GEO accession numbers. All experiments from which data used in this study were obtained were performed in mice (Mus musculus). Expression values obtained using different probes of DYNLL1 were normalized based on mean expression values obtained with each probe, and then, all the data were combined. In plots, different colors of data points indicate different probes used in the microarray. GEO profiles of datasets were given in figure captions.
Data analysis and visualization
Data analysis and visualization was performed in R statistical programming environment in this study (R version 4.0.2 (2020-06-22)) (R Core Team, 2020). Following R packages were used throughout the analysis: readxl (Wickham & Bryan, 2019), tidyverse (Wickham et al., 2019), ggpubr (Kassambara, 2020), knitr (Xie, 2021), and rmarkdown (Allaire et al., 2021; Xie et al., 2020).
Following convention for star symbols indicating statistical significance (P values) was used in the analysis of data in the present study: non-significant (ns): P > 0.05; *: P ≤ 0.05; **: P ≤ 0.01; ***: P ≤ 0.001;****: P ≤ 0.0001 (Kassambara, 2020). The normality test for the data used in each plot was performed using ggqqplot() (Quantile–Quantile plot) and shapiro.test() (Shapiro–Wilk normality test) functions in R (from ggpubr and stats R packages) (Kassambara, 2020; R Core Team, 2020). When data are normally distributed, we used Student’s t test to compare group means; otherwise, we performed the analysis using two-sample Wilcoxon test (Mann–Whitney test) due to high variance of transcript levels.
R script written to analyze the data can be found in supplementary documents to make the study completely reproducible.
Results
DYNLL1 transcript abundance decreases gradually during oocyte and follicular growth in mice
We first analyzed the changes in DYNLL1 transcript abundance during oocyte development from primordial follicle stage to large antral follicle stage in mice, using a publicly available transcript profiling dataset (GSE3351) (Fig. 1) (Pan et al., 2005). Authors of this dataset analyzed oocytes collected from ovaries of B6SJLF1 mice at 2 to 22 days of age (oocytes were isolated from primordial, primary, secondary, small antral, and large antral follicles obtained from animals that were 2, 6, 12, 17, and 22 days old, respectively) (Pan et al., 2005). Here, we should note that expression data are within oocytes from each stage of development, and not within whole follicles (Pan et al., 2005). We found that DYNLL1 mRNA levels decrease gradually with each stage of follicular growth (Fig. 1). For instance, DYNLL1 mRNA transcript levels in primordial follicle stage are more than threefold higher than that in large antral follicle stage, the last stage of follicular growth (Fig. 1, P = 7.4e-07). Mean normalized DYNLL1 expression values for each stage during oocyte and follicular growth are as follows: primordial follicle stage = 163; primary follicle stage = 127; secondary follicle stage = 96.0; small antral follicle stage = 62; and large antral follicle stage = 52.2 (Fig. 1). It is clear from these data that DYNLL1 mRNA levels decline dramatically with each subsequent stage of follicular growth in mice.
Fig. 1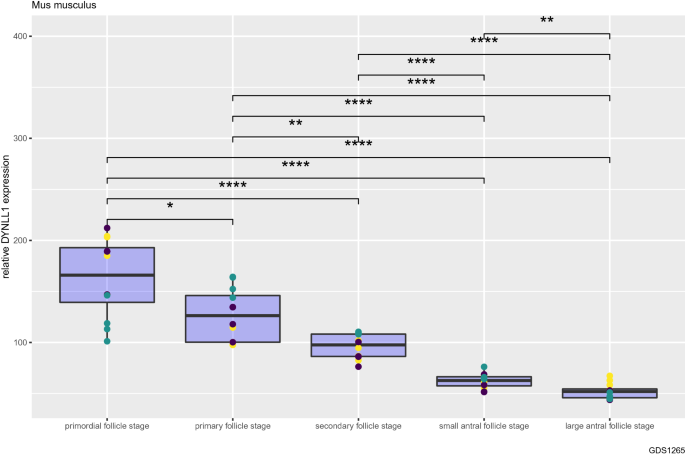
DYNLL1 transcript abundance decreases gradually during oocyte and follicular growth in mice. Relative DYNLL1 transcript abundance from primordial follicle stage (non-growing or dormant) to large antral follicle stage. Different colors of data points indicate different probes used in the microarray. Non-significant (ns): non-significant (ns): P > 0.05; *: P ≤ 0.05; **: P ≤ 0.01; ***: P ≤ 0.001;****: P ≤ 0.0001. Data from GSE3351 (n = 60)
Full size imageConsidering the role of DYNLL1 in DNA double-strand break (DSB) repair choice (it promotes NHEJ repair and in contrast limits accurate and error-free HR repair), we compared expression of certain genes functioning in HR and NHEJ DNA repair pathways during oocyte and follicular growth. We found that HR genes (BRCA1, BRCA2 and RAD51) generally show a trend of increasing expression from primordial follicle stage to large antral follicle stage (Supplementary Fig. 1). In contrast, the expression of NHEJ genes (XRCC5 and XRCC6) is the highest in the primordial follicle stage (Supplementary Fig. 1).
DYNLL1 transcript abundance decreases during oocyte meiotic maturation in mice
Next, we compared DYNLL1 mRNA transcript levels during meiotic maturation of the oocyte from immature germinal vesicle (GV) stage to mature metaphase II (MII) stage using two independent mouse gene expression datasets (GSE5668, GSE11667) (Fig. 2) (Pan et al., 2008; Salisbury et al., 2009; Su et al., 2007). We found that in the course of meiotic maturation, DYNLL1 transcript abundance decreases significantly in oocytes (Fig. 2). We also observed that this change in DYNLL1 mRNA levels from immature GV stage to mature MII stage is independent of the age of the animal, since we observed the same trend for both young (6–12 weeks of age) and old mice (60–70 weeks of age) (Fig. 2, second plot, two subplots) (Pan et al., 2008). In other words, it can be stated that even in old mice, DYNLL1 mRNA levels decrease from immature GV stage to mature MII stage (Fig. 2, second plot, last panel).
Fig. 2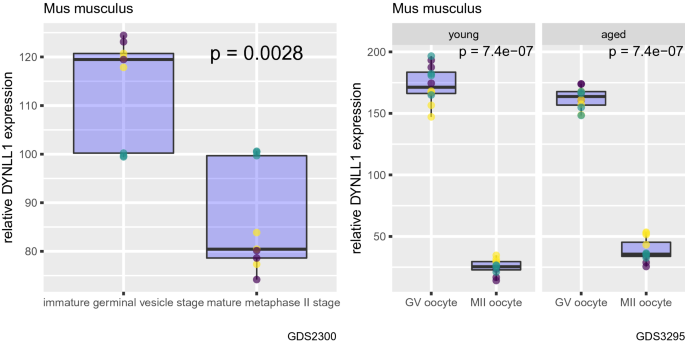
DYNLL1 transcript abundance decreases during oocyte meiotic maturation in mice. Changes in DYNLL1 transcript abundance from immature germinal vesicle (GV) stage to mature metaphase II (MII) stage. In the second plot, first subplot shows data from young mice, second subplot shows data from aged mice. Different colors of data points indicate different probes used in the microarray
Full size imageSimilarly, expression of NHEJ genes (XRCC5 and XRCC6) is higher in immature GV stage oocytes compared with mature MII stage oocytes (Supplementary Fig. 2).
Human chorionic gonadotropin (hCG) decreases DYNLL1 mRNA transcript levels in cumulus oocyte complexes in mice
Then, we investigated how treatment with human chorionic gonadotropin (hCG) (which induces ovulation) affects DYNLL1 mRNA transcript levels in cumulus oocyte complexes (COCs) isolated from mouse ovaries in which follicular growth was stimulated using equine chorionic gonadotropin (eCG) (Fig. 3) (Hernandez-Gonzalez et al., 2006). Authors of the dataset analyzed in the current study originally aimed to identify candidate genes that impact ovulation in cumulus oocyte complexes in mice (GSE4260) (Hernandez-Gonzalez et al., 2006). By using the same dataset, we observed that 16 h, but not 8 h, treatment with hCG results in a significant decrease in DYNLL1 levels (Fig. 3, P = 0.00054). This indicates that DYNLL1 levels might differ between before and after ovulation.
Fig. 3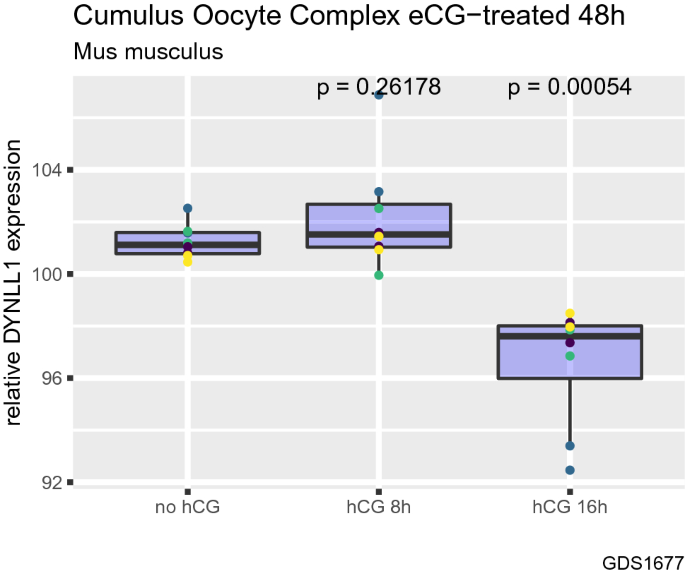
Human chorionic gonadotropin (hCG) decreases DYNLL1 mRNA transcript levels in cumulus oocyte complexes in mice. The effect of treatment with human chorionic gonadotropin (hCG) which induce ovulation on DYNLL1 mRNA transcript levels in cumulus oocyte complexes (COCs) isolated from mouse ovaries in which follicular growth was stimulated using equine chorionic gonadotropin (eCG). Different colors of data points indicate different probes used in the microarray
Full size imageOvaries from high-fertility mice have decreased DYNLL1 transcript abundance compared with that of control mice
Finally, we compared DYNLL1 mRNA levels between ovaries from high-fertility mice which exhibit an increase in ovulation number and ovaries from control mice using a dataset by Vanselow et al. (2008) (GSE11113) (Fig. 4). We found that high-fertility mice have lower DYNLL1 mRNA levels compared with control mice (Fig. 4, P = 2.4e-15). In other words, mice with higher ovulation numbers have decreased DYNLL1 mRNA transcript levels, somewhat in parallel to data presented in Fig. 3.
Fig. 4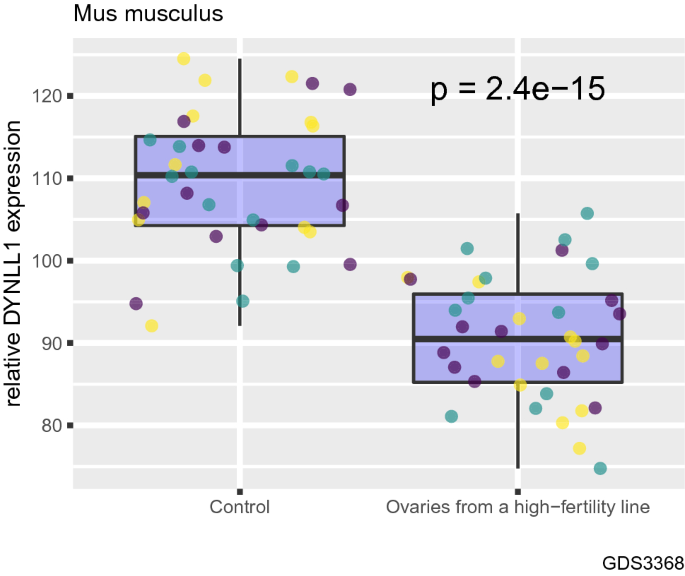
Ovaries from high-fertility mice have decreased DYNLL1 transcript abundance compared with that of control mice. Comparison of DYNLL1 mRNA levels between ovaries from high-fertility mice which exhibit an increase in ovulation number and ovaries from control mice. Different colors of data points indicate different probes used in the microarray
Full size imageThe expression of XRCC6, a NHEJ gene, is lower in high-fertility mice compared with control mice, similar to what was observed with DYNLL1 (Supplementary Fig. 3).
Discussion
Here, we showed that DYNLL1 transcript abundance decreases during oocyte and follicular growth, and during oocyte meiotic maturation in mice. DYNLL1 is known to promote error-prone non-homologous end joining (NHEJ) repair and in contrast to limit error-free homologous recombination (HR) repair; thus, it participates in DSB repair choice (Becker et al., 2018; Berkel & Cacan, 2021; He et al., 2018; West et al., 2019). Therefore, its decreased cellular levels during oocyte and follicular growth might be associated with increased genomic stability due to relatively increased HR repair and decreased NHEJ repair events (Becker et al., 2018; He et al., 2018; West et al., 2019). Since the oocytes in the non-growing primordial follicles are arrested in the last stage of prophase and do not undergo further mitotic division until sexual maturity, these oocytes might have increased NHEJ repair events and decreased HR repair events due to their higher DYNLL1 levels (Clarke, 2017). However, during reproductive life, these non-growing or dormant primordial follicles begin to proliferate mitotically and to be recruited into the growing pool; thus, they may gradually decrease their DYNLL1 levels in the course of follicular growth to promote error-free HR repair over error-prone NHEJ repair (Clarke, 2017; Da Silva-Buttkus et al., 2008; Hirshfield, 1991; Lee & Chang, 2019; Wright et al., 2020). This is in line with the observation that the choice of DSB repair pathway is dependent on the cell cycle phases, and that mitotic cells can repair DSBs via a homology-directed pathway, a major pathway used for HR repair (Sakamoto et al., 2021). In contrast, Stringer et al. showed that around 90% of prophase-arrested oocytes in primordial follicles exposed to γ-irradiation utilized HR repair pathway and the remaining 10% utilized the NHEJ repair pathway (Newman et al., 2022). However, somatic granulosa cells surrounding oocytes in these follicles primarily utilized NHEJ repair pathway (Newman et al., 2022). Therefore, it might be stated that oocytes in primordial follicles utilize mostly HR repair pathway, even though they have higher DYNLL1 levels. Supporting our findings, authors reported that most of the non-growing prophase-arrested oocytes in primordial follicles exposed to even low doses of γ-irradiation died; however, growing follicles remained largely unaffected, pointing out the unique sensitivity of non-growing prophase-arrested oocytes in primordial follicles compared with oocytes in growing follicles (Newman et al., 2022). Considering that the gamma radiation leads to dose-dependent increases in DNA damage, these differences in sensitivity between growing follicles and primordial follicles might be due to, at least to a certain extent, lower DYNLL1 levels in oocytes in growing follicles (thus even higher HR repair events) compared with oocytes in primordial follicles, although primary DSB repair choice in oocytes in primordial follicles is HR repair. However, these differences in sensitivity might also be attributed to the differences in the expression of key regulators of cell death.
Although they did not study DYNLL1, Horta et al. showed that transcript abundance of most other DNA repair genes is lower in MII oocytes compared with GV oocytes in young mice, in parallel to our findings showing that DYNLL1 transcript abundance decreases during oocyte meiotic maturation from GV stage to MII stage in mice (Horta et al., 2021). They observed that in old mice, transcript abundance of some of the DNA repair genes increases during the GV–MII transition, showing the effect of age on relative transcript abundance of DNA repair genes in GV-to-MII transition in mice. However, we observed that DYNLL1 mRNA transcript levels decrease in both young and aged mice from immature GV stage to mature MII stage. Therefore, considering that the age is an important parameter in terms of women reproductive health and it has a major impact in oocyte quality and reserve, changes in the cellular levels and activities of DNA repair proteins should be studied in more detail to better understand age-dependent changes in genomic stability in oocytes. These studies will ultimately have the potential to improve clinical success rates of assisted reproductive technologies in older women by helping to develop novel strategies to increase genomic stability in their oocytes (Horta et al., 2020; Newman et al., 2022).
We also found that ovaries from high-fertility mice with increased ovulation numbers have decreased DYNLL1 transcript abundance compared with that of control mice, and the induction of ovulation with human chorionic gonadotropin (hCG) results in a decrease in DYNLL1 mRNA transcript levels in cumulus oocyte complexes isolated from mouse ovaries. In other words, DYNLL1 levels might be inversely associated with fertility and ovulation in mice. This might be due to increased number of error-free HR repair events in ovaries and oocytes with lower DYNLL1 levels as detailed above. As it is shown that DNA repair is sufficient to restore functional fertility, and DNA repair may be an important mechanism for ensuring female fertility, higher HR repair preference due to lower DYNLL1 levels might be a contributing factor to the higher fertility observed in mice with decreased DYNLL1 levels (Stringer et al., 2018, 2020; Horta et al., 2021; Winship et al., 2018).
Furthermore, a decrease in DYNLL1 transcript abundance during in vitro oocyte maturation from GV stage to MII stage was also reported in bovine oocytes, showing that these changes might not be species-specific and may point to some universal roles of DYNLL1 in oocyte development in diverse taxa (Racedo et al., 2008). Further research is needed to make stronger inferences.
Conclusion
In conclusion, we hypothesize that DYNLL1 might be an important player during oocyte and follicular growth possibly by regulating the number of HR repair events, and that decreased DYNLL1 levels might contribute to fertility by promoting HR repair over NHEJ repair and ultimately by increasing genomic stability. Further in vivo and mechanistic studies are required to confirm our findings to better understand the role of DYNLL1 in oocyte and follicle development and its association with fertility to improve reproductive performance, to slow down the depletion of ovarian follicle reserve, and to delay menopause. More data are needed to clearly and precisely show that DYNLL1 has important mechanistic roles in oocytes and follicles, or in general in ovaries. As the limitations of our study, we performed all the analysis at the mRNA transcript level due to availability of transcriptomics data; therefore, further analysis at the protein level will also be highly informative. The functionality of DYNLL1 in oocytes also has not been previously studied and its functions other than those in DNA repair might be of high importance such as its role as an important inhibitor of nitric oxide synthase which regulates many biological processes in the ovary including oocyte maturation and ovulation (Thaler & Epel, 2003). DYNLL1 has also recently been highlighted as having a key role in regulating the primordial to primary follicle transition (Hamazaki et al., 2021). Therefore, decreases in DYNLL1 transcript abundance during oocyte and follicular growth might possibly lead to molecular changes in these pathways in addition to DNA damage.
Data availability
Datasets used in the present study were given in Materials and methods section.
References
Adhikari, D., & Liu, K. (2014). The regulation of maturation promoting factor during prophase I arrest and meiotic entry in mammalian oocytes. Molecular and Cellular Endocrinology, 382(1), 480–487. https://doi.org/10.1016/j.mce.2013.07.027. Epub 2013 Aug 3 PMID: 23916417.
Allaire, J. J., Xie, Y., McPherson, J., Luraschi, J., Ushey, K., Atkins, A., Wickham, H., Cheng, J., Chang, W., & Iannone, R. (2021). rmarkdown: Dynamic Documents for R. R package version 2.10. https://rmarkdown.rstudio.com.
Becker, J. R., Cuella-Martin, R., Barazas, M., Liu, R., Oliveira, C., Oliver, A. W., Bilham, K., Holt, A. B., Blackford, A. N., Heierhorst, J., Jonkers, J., Rottenberg, S., & Chapman, J. R. (2018). The ASCIZ-DYNLL1 axis promotes 53BP1-dependent non-homologous end joining and PARP inhibitor sensitivity. Nature Communications, 9(1), 5406. https://doi.org/10.1038/s41467-018-07855-x.PMID:30559443;PMCID:PMC6297349
Berkel, C., & Cacan, E. (2020). In silico analysis of DYNLL1 expression in ovarian cancer chemoresistance. Cell Biology International, 44(8), 1598–1605. https://doi.org/10.1002/cbin.11352. Epub 2020 Apr 13 PMID: 32208526.
Berkel, C., & Cacan, E. (2021). Involvement of ATMIN-DYNLL1-MRN axis in the progression and aggressiveness of serous ovarian cancer. Biochemical and Biophysical Research Communications, 17(570), 74–81. https://doi.org/10.1016/j.bbrc.2021.07.004. Epub 2021 Jul 14 PMID: 34273621.
Clarke, H. (2017). Control of mammalian oocyte development by interactions with the maternal follicular environment. Results and Problems in Cell Differentiation, 63, 17–41. https://doi.org/10.1007/978-3-319-60855-6_2. PMID: 28779312.
Conti, M., Hsieh, M., Zamah, A. M., & Oh, J. S. (2012). Novel signaling mechanisms in the ovary during oocyte maturation and ovulation. Molecular and Cell Endocrinology, 356(1–2), 65–73. https://doi.org/10.1016/j.mce.2011.11.002. Epub 2011 Nov 12 PMID: 22101318.
Coticchio, G., Dal Canto, M., Mignini Renzini, M., Guglielmo, M. C., Brambillasca, F., Turchi, D., Novara, P. V., & Fadini, R. (2015). Oocyte maturation: gamete-somatic cells interactions, meiotic resumption, cytoskeletal dynamics and cytoplasmic reorganization. Human Reproduction Update, 21(4), 427–454. https://doi.org/10.1093/humupd/dmv011. Epub 2015 Mar 4 PMID: 25744083.
Da Silva-Buttkus, P., Jayasooriya, G. S., Mora, J. M., Mobberley, M., Ryder, T. A., Baithun, M., Stark, J., Franks, S., & Hardy, K. (2008). Effect of cell shape and packing density on granulosa cell proliferation and formation of multiple layers during early follicle development in the ovary. Journal of Cell Science, 121(Pt 23), 3890–3900. https://doi.org/10.1242/jcs.036400. Epub 2008 Nov 11 PMID: 19001500.
Faddy, M. J., Gosden, R. G., Gougeon, A., Richardson, S. J., & Nelson, J. F. (1992). Accelerated disappearance of ovarian follicles in mid-life: Implications for forecasting menopause. Human Reproduction, 7, 1342–1346.
Hamazaki, N., Kyogoku, H., Araki, H., Miura, F., Horikawa, C., Hamada, N., Shimamoto, S., Hikabe, O., Nakashima, K., Kitajima, T. S., Ito, T., Leitch, H. G., & Hayashi, K. (2021). Reconstitution of the oocyte transcriptional network with transcription factors. Nature, 589(7841), 264–269. https://doi.org/10.1038/s41586-020-3027-9. Epub 2020 Dec 16 PMID: 33328630.
Hansen, K. R., Knowlton, N. S., Thyer, A. C., Charleston, J. S., Soules, M. R., & Klein, N. A. (2008). A new model of reproductive aging: The decline in ovarian non-growing follicle number from birth to menopause. Human Reproduction, 23, 699–708.
He, Y. J., Meghani, K., Caron, M. C., Yang, C., Ronato, D. A., Bian, J., Sharma, A., Moore, J., Niraj, J., Detappe, A., Doench, J. G., Legube, G., Root, D. E., D’Andrea, A. D., Drané, P., De, S., Konstantinopoulos, P. A., Masson, J. Y., & Chowdhury, D. (2018). DYNLL1 binds to MRE11 to limit DNA end resection in BRCA1-deficient cells. Nature, 563(7732), 522–526. https://doi.org/10.1038/s41586-018-0670-5. Epub 2018 Oct 31 PMID: 30464262.
Hernandez-Gonzalez, I., Gonzalez-Robayna, I., Shimada, M., Wayne, C. M., et al. (2006). Gene expression profiles of cumulus cell oocyte complexes during ovulation reveal cumulus cells express neuronal and immune-related genes: Does this expand their role in the ovulation process? Molecular Endocrinology, 20(6), 1300–1321. PMID: 16455817.
Hirshfield, A. N. (1991). Development of follicles in the mammalian ovary. International Review of Cytology, 124, 43–101. https://doi.org/10.1016/s0074-7696(08)61524-7. PMID: 2001918.
Holt, J. E., Lane, S. I., & Jones, K. T. (2013). The control of meiotic maturation in mammalian oocytes. Current Topics in Developmental Biology, 102, 207–226. https://doi.org/10.1016/B978-0-12-416024-8.00007-6. PMID: 23287034.
Horta, F., Catt, S., Ramachandran, P., Vollenhoven, B., & Temple-Smith, P. (2020). Female ageing affects the DNA repair capacity of oocytes in IVF using a controlled model of sperm DNA damage in mice. Human Reproduction, 35(3), 529–544.
Horta, F., Ravichandran, A., Catt, S., Vollenhoven, B., & Temple-Smith, P. (2021). Ageing and ovarian stimulation modulate the relative levels of transcript abundance of oocyte DNA repair genes during the germinal vesicle-metaphase II transition in mice. Journal of Assisted Reproduction and Genetics, 38(1), 55–69. https://doi.org/10.1007/s10815-020-01981-6. Epub 2020 Oct 17 PMID: 33067741.
Kassambara, A. (2020). ggpubr: 'ggplot2' Based Publication Ready Plots. R package version 0.4.0. https://CRAN.R-project.org/package=ggpubr
Lee, H. N., & Chang, E. M. (2019). Primordial follicle activation as new treatment for primary ovarian insufficiency. Clinical and Experimental Reproductive Medicine, 46(2), 43–49. https://doi.org/10.5653/cerm.2019.46.2.43. Epub 2019 Jun 1 PMID: 31181871.
Newman, H., Catt, S., Vining, B., Vollenhoven, B., & Horta, F. (2022). DNA repair and response to sperm DNA damage in oocytes and embryos, and the potential consequences in ART: a systematic review. Molecular Human Reproduction, 28(1), gaab071.
Pan, H., Ma, P., Zhu, W., & Schultz, R. M. (2008). Age-associated increase in aneuploidy and changes in gene expression in mouse eggs. Developmental Biology, 316(2), 397–407. PMID: 18342300.
Pan, H., O’brien, M. J., Wigglesworth, K., Eppig, J. J., & Schultz, R. M. (2005). Transcript profiling during mouse oocyte development and the effect of gonadotropin priming and development in vitro. Developmental Biology, 286(2), 493–506. PMID: 16168984.
R Core Team. (2020). R: A Language and Environment for Statistical Computing. Vienna, Austria: R Foundation for Statistical Computing. https://www.R-project.org/.
Racedo, S. E., Wrenzycki, C., Herrmann, D., Salamone, D., & Niemann, H. (2008). Effects of follicle size and stages of maturation on mRNA expression in bovine in vitro matured oocytes. Molecular Reproduction and Development, 75(1), 17–25. https://doi.org/10.1002/mrd.20770. PMID: 17546584.
Sakamoto, Y., Kokuta, T., Teshigahara, A., Iijima, K., Kitao, H., Takata, M., & Tauchi, H. (2021). Mitotic cells can repair DNA double-strand breaks via a homology-directed pathway. Journal of Radiation Research, 62(1), 25–33. https://doi.org/10.1093/jrr/rraa095.PMID:33009557;PMCID:PMC7779344
Salisbury, J., Hutchison, K. W., Wigglesworth, K., Eppig, J. J., et al. (2009). Probe-level analysis of expression microarrays characterizes isoform-specific degradation during mouse oocyte maturation. PLoS ONE, 4(10), e7479. PMID: 19834616.
Stringer, J. M., Winship, A., Liew, S. H., & Hutt, K. (2018). The capacity of oocytes for DNA repair. Cellular and Molecular Life Sciences, 75(15), 2777–2792. https://doi.org/10.1007/s00018-018-2833-9. Epub 2018 May 10 PMID: 29748894.
Stringer, J. M., Winship, A., Zerafa, N., Wakefield, M., & Hutt, K. (2020). Oocytes can efficiently repair DNA double-strand breaks to restore genetic integrity and protect offspring health. Proceedings of the National Academy of Sciences of the United States of America, 117(21), 11513–11522. https://doi.org/10.1073/pnas.2001124117. Epub 2020 May 7 PMID: 32381741.
Su, Y. Q., Sugiura, K., Woo, Y., Wigglesworth, K., et al. (2007). Selective degradation of transcripts during meiotic maturation of mouse oocytes. Developmental Biology, 302(1), 104–117. PMID: 17022963.
Thaler, C. D., & Epel, D. (2003). Nitric oxide in oocyte maturation, ovulation, fertilization, cleavage and implantation: A little dab’ll do ya. Current Pharmaceutical Design, 9(5), 399–409. https://doi.org/10.2174/1381612033391748. PMID: 12570817.
Titus, S., Li, F., Stobezki, R., Akula, K., Unsal, E., Jeong, K., Dickler, M., Robson, M., Moy, F., Goswami, S., & Oktay, K. (2013). Impairment of BRCA1-related DNA double-strand break repair leads to ovarian aging in mice and humans. Science Translational Medicine, 5(172), 172ra21. https://doi.org/10.1126/scitranslmed.3004925.PMID:23408054;PMCID:PMC5130338
Vanselow, J., Nürnberg, G., Koczan, D., Langhammer, M., et al. (2008). Expression profiling of a high-fertility mouse line by microarray analysis and qPCR. BMC Genomics, 27(9), 307. PMID: 18588666.
West, K. L., Kelliher, J. L., Xu, Z., An, L., Reed, M. R., Eoff, R. L., Wang, J., Huen, M. S. Y., & Leung, J. W. C. (2019). LC8/DYNLL1 is a 53BP1 effector and regulates checkpoint activation. Nucleic Acids Research, 47(12), 6236–6249. https://doi.org/10.1093/nar/gkz263.PMID:30982887;PMCID:PMC6614850
Wickham, H., & Bryan, J. (2019). readxl: Read Excel Files. R package version 1.3.1. https://CRAN.R-project.org/package=readxl.
Wickham, H., et al. (2019). Welcome to the tidyverse. Journal of Open Source Software, 4(43), 1686. https://doi.org/10.21105/joss.01686.
Winship, A. L., Stringer, J. M., Liew, S. H., & Hutt, K. J. (2018). The importance of DNA repair for maintaining oocyte quality in response to anti-cancer treatments, environmental toxins and maternal ageing. Human Reproduction Update, 24(2), 119–134. https://doi.org/10.1093/humupd/dmy002. PMID: 29377997.
Wright, C. J., Cari, E. L., Sandoval, J., Bales, E., Sam, P. K., Zarate, M. A., Polotsky, A. J., Kallen, A. N., & Johnson, J. (2020). Control of murine primordial follicle growth activation by IκB/NFκB signaling. Reproductive Sciences, 27(11), 2063–2074. https://doi.org/10.1007/s43032-020-00225-3. Epub 2020 Jun 15 PMID: 32542534.
Xie, Y. (2021). knitr: A General-Purpose Package for Dynamic Report Generation in R. R package version 1.34.
Xie, Y., Dervieux, C., & Riederer, E. (2020). R Markdown Cookbook. Chapman and Hall/CRC. ISBN 9780367563837. https://bookdown.org/yihui/rmarkdown-cookbook.
Younis, J. S. (2011). Ovarian aging: Latest thoughts on assessment and management. Current Opinion in Obstetrics and Gynecology, 23, 427–434.
Funding
CB is funded by TUBITAK (The Scientific and Technological Research Council of Turkey) 2211-E grant.
Author information
Authors and Affiliations
Department of Molecular Biology and Genetics, Tokat Gaziosmanpasa University, Tokat, Turkey
Caglar Berkel & Ercan Cacan
Corresponding authors
Correspondence to Caglar Berkel or Ercan Cacan.
Ethics declarations
Conflict of interest
Authors declare that they have no conflict of interest.
Supplementary Information
Below is the link to the electronic supplementary material.
Supplementary file1 (PDF 13 KB)
Supplementary file2 (PDF 6 KB)
Supplementary file3 (PDF 6 KB)
Rights and permissions
Springer Nature or its licensor (e.g. a society or other partner) holds exclusive rights to this article under a publishing agreement with the author(s) or other rightsholder(s); author self-archiving of the accepted manuscript version of this article is solely governed by the terms of such publishing agreement and applicable law.
About this article
Cite this article
Berkel, C., Cacan, E. DYNLL1 transcript abundance decreases during oocyte and follicular growth and is associated with fertility in mice. GENOME INSTAB. DIS. (2022). https://doi.org/10.1007/s42764-022-00096-9
Received06 October 2022
Revised20 November 2022
Accepted29 November 2022
Published06 December 2022
DOIhttps://doi.org/10.1007/s42764-022-00096-9
Share this article
Anyone you share the following link with will be able to read this content:
Get shareable linkKeywords
Oocyte
DNA repair
DYNLL1
Fertility
Oocyte reserve
Follicular growth
Ovary
NHEJ
Homologous recombination
Genome stability
用户登录
还没有账号?
立即注册