An integrated pan-cancer analysis of leucine-rich repeat containing protein 59: a potential biomarker for prognostic and immunotherapy
Original Research Paper
Published: 21 November 2023
Meiqi Zeng, Xia Wang, Xiaona Wang, Yuning Zhang, Zhenguang Ying, Lixin Xia, Feng Gao, Xianxiong Chen, Kin Yip Tam, Long Xu & Ou Sha
Genome Instability & Disease Volume 4, pages 333–348, (2023)
Abstract
Leucine-rich repeat containing protein 59 (LRRC59), a ribosome binding protein located on the endoplasmic reticulum and the nuclear envelope. Although it has been found in blood plasma and linked to the development of a few cancers, the function of LRRC59 is still largely unknown and there is no systematic investigation on its role in various human cancers. We performed a multi-omics data analysis to investigate the expression of LRRC59 in human tumors and its correlation with clinical prognosis, gene set enrichment, mutation status, and immune infiltration in cancers using the TCGA, GTEx, GEPIA2, HPA, UALCAN, Timer2, GTBAdb, cBioPortal database and R packages. In the majority of TCGA tumors, LRRC59 was expressed significantly differently (up-regulated in 25 and down-regulated in 4 cancer types). High LRRC59 expression has been linked to worse prognosis in several malignancies. In numerous carcinomas, the expression of LRRC59 was associated clinicopathological stages. The LRRC59 regulation network was mainly involved in the pathways related to endoplasmic reticulum homeostatic and cell proliferation. In addition, the expression of LRRC59 is also strongly associated with the immune cell infiltration. LRRC59 could also predicts the response to immunotherapy. LRRC59 is a potential valuable biomarker not only for diagnostic and prognostic, but also for immunotherapy in most cancers.
Introduction
Cancer is a global public health concern and the second leading cause of mortality in the United States (Siegel et al., 2022). As a result of significant improvements in diagnosis and cutting-edge therapy options, cancer death rates decreased, yet it remains a global hazard to human health. A common mechanism or target that may be exploited to treat all malignancies is what researchers are searching for. Pan-cancer analysis, which makes use of a number of open-access data sets, is an effective technique for identifying new oncogenes and expanding the universe of potential treatments. We first and thoroughly analyzed the expression profiles and prognostic value of leucine-rich repeat containing protein 59 (LRRC59) in diverse cancers to uncover novel biomarkers and create new treatments.
LRRC59, a type II transmembrane protein with a short C-terminal domain, has been reported to be closely related to the development of human malignancies. For instance, elevated expression level of LRRC59 is associated with poor prognosis in lung cancer, head and neck squamous cell carcinoma, breast cancer and urothelial carcinoma (UC) (Geng et al., 2021; Li et al., 2020; Pei et al., 2022; Toda et al., 2018). Knockdown of LRRC59 significantly inhibited UC cell proliferation, migration and resulted in cell cycle arrest, while over-expressing LRRC59 in UC cells enhanced cell proliferation and migration (Li et al., 2020). The fusion transcript formed by LRRC59 and FLJ60017 is specific to predict prostate cancer recurrence (Yu et al., 2014). Downstream of HMGA1, LRRC59 is also demonstrated to be involved in the modulation of cell motility of breast cancer (Maurizio et al., 2016). Localized on endosome and nuclear membrane, LRRC59 is critical for the nuclear transportation of exogenous FGF1, a key gene in the progression of cancer (Zhen et al., 2012). The oncoprotein Cancerous Inhibitor of PP2A (CIP2A), a tumor suppressor, interacts with LRRC59 for the nuclear import and proper function (Pallai et al., 2015). In addition, LRRC59 is important in nuclear transport and Toll-like receptor translocation during viral infection (Tatematsu et al., 2015; Zhen et al., 2012). These processes are crucial for the activation of innate immune responses to viral pathogens. During viral infection, LRRC59 is also significant in promoting the activation of type I interferon (IFN) signaling, which plays a vital role in antiviral defense by inducing an antiviral state in infected and neighboring cells (Xian et al., 2020).
Despite the fact that LRRC59 has multiple roles in a few cancers, not enough study has been conducted to determine how it links to the clinical and immunological aspects of pan-cancer. Based on a multiomic bioinformatic analysis, we explored the relationships between LRRC59 expression and immune signature enrichment, progression features, and clinical outcomes in 33 cancer types from The Cancer Genome Atlas (TCGA). This study aims to provide novel insights into the role of LRRC59 in cancer biology and clinical practice. The flow chart is shown in Fig. 1.
Fig. 1
Workflow of this study
Materials and methods
Gene and protein expression analysis of LRRC59
Gene Expression Profiling Interactive Analysis 2 (GEPIA2) database (http://gepia2.cancer-pku.cn/#index) was utilized to investigate the median expression of LRRC59 in tumor and normal tissue samples. The Human Protein Atlas (HPA) database (https://www.proteinatlas.org) was used to reveal the protein concentration of LRRC59 in blood and the mRNA expression of LRRC59 at the cellular level. TCGA database contained sequencing data from different tumor tissues. The Genotype-Tissue Expression (GTEx) database contained information on normal human tissue data (Vivian et al., 2017). The UCSC Xena website (https://xenabrowser.net/datapages/) was used to retrieve the transcripts per million (TPM) format Toil TCGA and GTEx RNA-seq recompute data. These data were converted to log2 format. Using the Wilcoxon rank-sum test, normal and tumor samples (representing 33 different forms of cancer) were analyzed and compared. The CPTAC data set of UALCAN database (http://ualcan.path.uab.edu) was used to compare the protein expression of LRRC59 in different tumor and normal tissues (Chen et al., 2019).
Clinicopathological staging correlation analysis
TCGA delivers RNA-seq data in HTSeq–FPKM format and clinical data (TNM stage and pathological stage). The data in FPKM format were first transformed to TPM format, then converted by log2 (TPM + 1), and analyzed in a one-way ANOVA.
Survival prognosis analysis
RNA-seq expression profiles and corresponding clinical information for LRRC59 were obtained from the TCGA. Using the univariate cox regression analysis, the importance of LRRC59 in predicting Overall Survival (OS) and Disease-Free Survival (DFS) in pan-cancer was determined. In addition, the OS and DFS significance map was collected using the “Survival Analysis” module of GEPIA2, then the Kaplan–Meier plots were generated selectively.
Immune cells infiltration analysis
The “Immune Association” module of Tumor Immune Estimation Resource version 2 (Timer2) (http://timer.cistrome.org/) database was used to analyze the correlations between LRRC59 expression and immune infiltrates in pan-cancer.
Immunotherapy prediction analysis
The TCGA RNA-seq data in HTSeq–FPKM format for 33 tumor types were obtained, then converted to TPM format and processed by log2 (TPM + 1). The connection between LRRC59 and 47 immunological checkpoint genes (ICGs) was determined using Spearman's rank correlation coefficients (Li et al., 2021). Spearman's rank correlation test was also employed to examine the associations between LRRC59 expression levels and tumor mutational burden (TMB) and microsatellite instability (MSI).
LRRC59-related gene enrichment analysis
The “panGTBA” module of GTBAdb (http://guotosky.vip:13838/GTBA/) database was utilized to analyze the link between the LRRC59 gene and the HALLMARK pathway.
Genetic alteration analysis
cBioPortal database (http://www.cbioportal.org/) was used to explore the genetic modification of LRRC59. A total of 10,967 samples (from 10,953 patients) were analyzed. “OncoPrint” module was used to analyze total mutations in pan-cancer, “Cancer Types Summary” module was used to determine the modification frequency of LRRC59 gene, “Mutations” module was used to investigate the mutation of LRRC59 in pan-cancer throughout protein domains. “Comparison/Survival” module was used to evaluate whether LRRC59 mutations are associated with patients’ OS, DFS, progression-free survival (PFS) and disease-specific survival (DSS).
LRRC59-related protein analysis
STRING database (https://cn.string-db.org/) was used to analyse the related proteins of LRRC59.
Statistical analysis and visualization
The R language software (version: 4.2.1) was applied for statistical analysis, and p values less than 0.05 were considered statistically significant (*p < 0.05, **p < 0.01, ***p < 0.001). The visualization results were created with the “ggplot2”, “forestplot” and “ggradar” R packages.
Results
LRRC59 was aberrantly expressed at both mRNA and protein levels in pan-cancer
Based on the interactive body map (Fig. 2a), we noticed that the median expression of LRRC59 differed between the majority of human tumor tissues and their corresponding normal tissues, specifically in the adrenal gland, blood, colon, testis, esophagus and stomach. The protein concentration of LRRC59 was detected in the plasma, the mean level was 740 ng/L (Fig. 2b). In addition, we observed the mRNA expression levels of LRRC59 in several human cell lines (Fig. 2c). We perceived that the expression levels of LRRC59 were higher in the brain, female reproductive system, mesenchyme, lymphoid and myeloid cell lines. LRRC59 was abnormally elevated in 25 cancer types (BLCA, BRCA, CESC, CHOL, COAD, DLBC, ESCA, GBM, HNSC, KIRC, KIRP, LGG, LIHC, LUAD, LUSC, OV, PAAD, PRAD, READ, SKCM, STAD, TGCT, THYM, UCEC, UCS), and down-regulated in 4 cancer types (ACC, KICH, LAML, PCPG) (Fig. 2d). Based on these previous findings, we further analyzed the protein expression of LRRC59 in 10 cancer types (Fig. 2e). Compared to normal samples, higher expression levels were observed in the tumor samples of BRCA, COAD, OV, KIRC, UCEC, LUAD, HNSC, GBM and LIHC, whereas PAAD was the only cancer type in which the expression level of LRRC59 decreased.
Fig. 2
LRRC59 gene expression in human pan-cancer. a Interactive BodyMap from GEPIA2 database. b Protein concentration of LRRC59 in blood. c Bar plot, the mRNA expression levels of LRRC59 in different cell lines based on HPA database. d mRNA expression status of LRRC59 in normal and tumor samples. e LRRC59 protein expression of normal and tumor samples was visualized using the UALCAN database. *p < 0.05, **p < 0.01, ***p < 0.001; ns not significant
LRRC59 was correlated with clinicopathological staging in partial cancer
We evaluated the expression of LRRC59 in cancer patients with stage I, II, III, IV and discovered that its expression was considerably elevated in ACC, LIHC, LUAD and STAD. There was no association between LRRC59 expression and the advancement of clinical staging in BLCA, BRCA, CHOL, COAD, ESCA, KICH, KIRC, KIRP, LUSC, PAAD, READ, SKCM, and TGCT (Fig. 3).
Fig. 3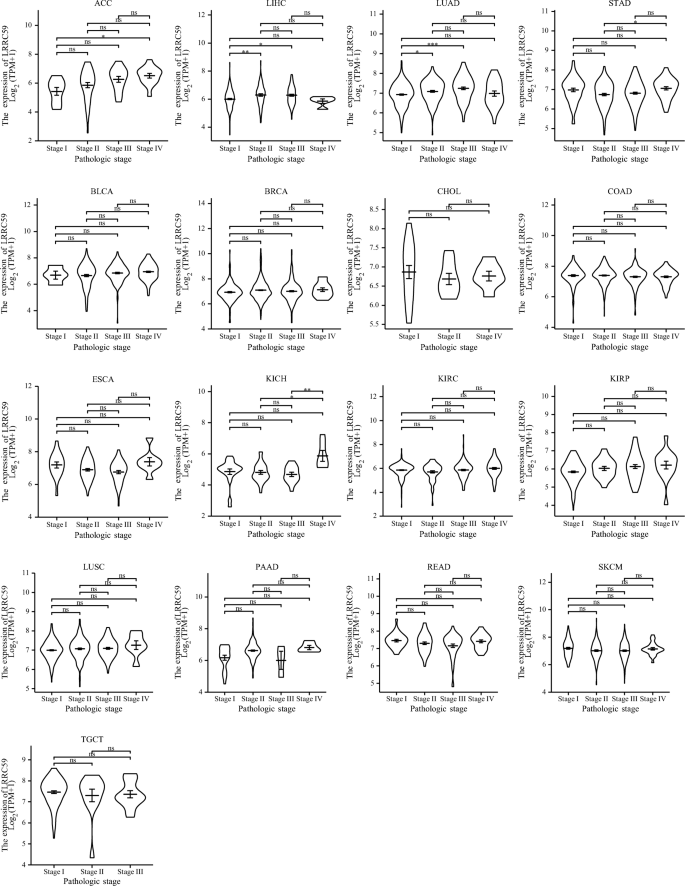
Correlations between LRRC59 expression and different pathological stages of pan-cancer. *p < 0.05, **p < 0.01, ***p < 0.001; ns not significant
LRRC59 was associated with survival prognosis in pan-cancer
We evaluated the prognostic value of LRRC59 in pan-cancer. Cox regression analysis was used to determine the relationship between LRRC59 expression level and prognostic markers, including OS and DFS (Fig. 4). The OS results revealed that LRRC59 was a risk factor for patients with ACC, BLCA, CESC, HNSC, KICH, LGG, LIHC, LUAD, MESO, PAAD, and UVM, but a protective factor for patients with COAD. The DFS results revealed that LRRC59 was a risk factor for patients with CESC and PAAD. The Kaplan–Meier survival curves and a survival significance map of LRRC59 in pan-cancer were created subsequently. Increased LRRC59 expression was solely linked with better OS in patients with COAD, whereas was associated with worse OS in patients with ACC, BLCA, HNSC, KICH, KIRP, LGG, LIHC, LUAD, MESO, SKCM and UVM (Fig. 5a). High expression of LRRC59 in patients with LAML was associated with a longer DFS, whereas low expression of LRRC59 in patients with ACC, CESC, LIHC, LUSC, PAAD and UVM was associated with a longer DFS (Fig. 5b). There was no statistical difference between the high and low expression levels of LRRC59 in the OS and DFS of other tumors.
Fig. 4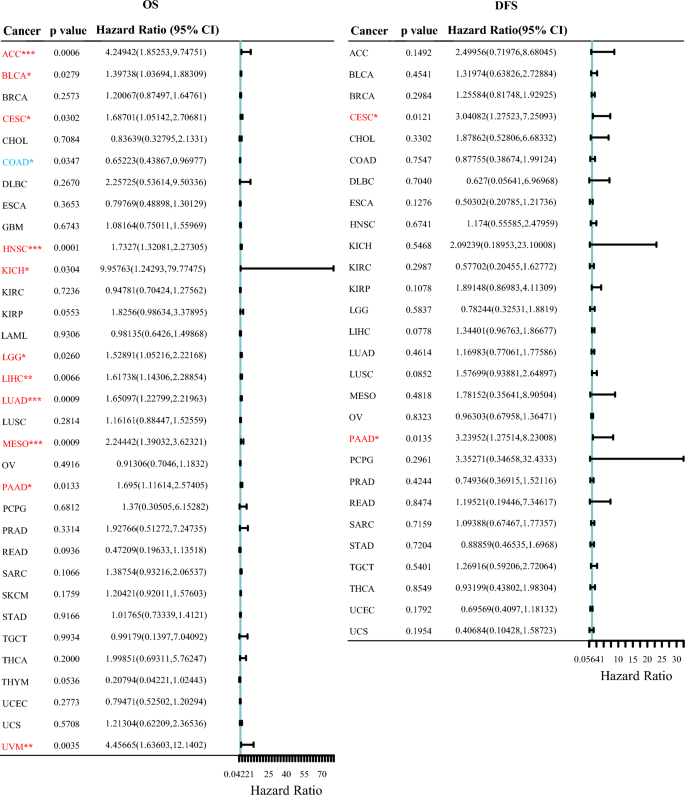
Univariate cox regression analysis of LRRC59 for OS and DFS. *p < 0.05, **p < 0.01, ***p < 0.001
Fig. 5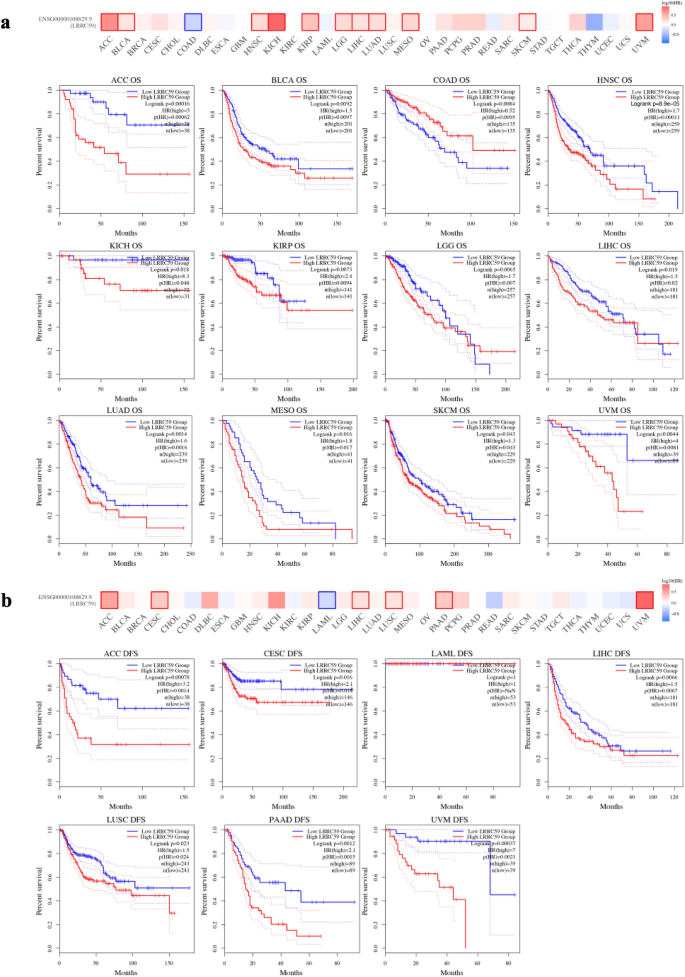
Associations between LRRC59 expression and survival prognosis in pan-cancer. a OS and b DFS analysis of LRRC59 using Kaplan–Meier from GEPIA2 database
LRRC59 was correlated with immune cells infiltration in pan-cancer
Analysis of immune infiltration demonstrated that the expression levels of LRRC59 correlated with the degree of immune infiltration in 33 cancer types. The results suggested that LRRC59 expression was negatively correlated with CD8+ T cell and activated mast cell infiltration levels, and favorably correlated with those of neutrophils, total macrophage, M1 macrophages, cancer-associated fibroblasts and endothelial cells (Fig. 6a–f).
Fig. 6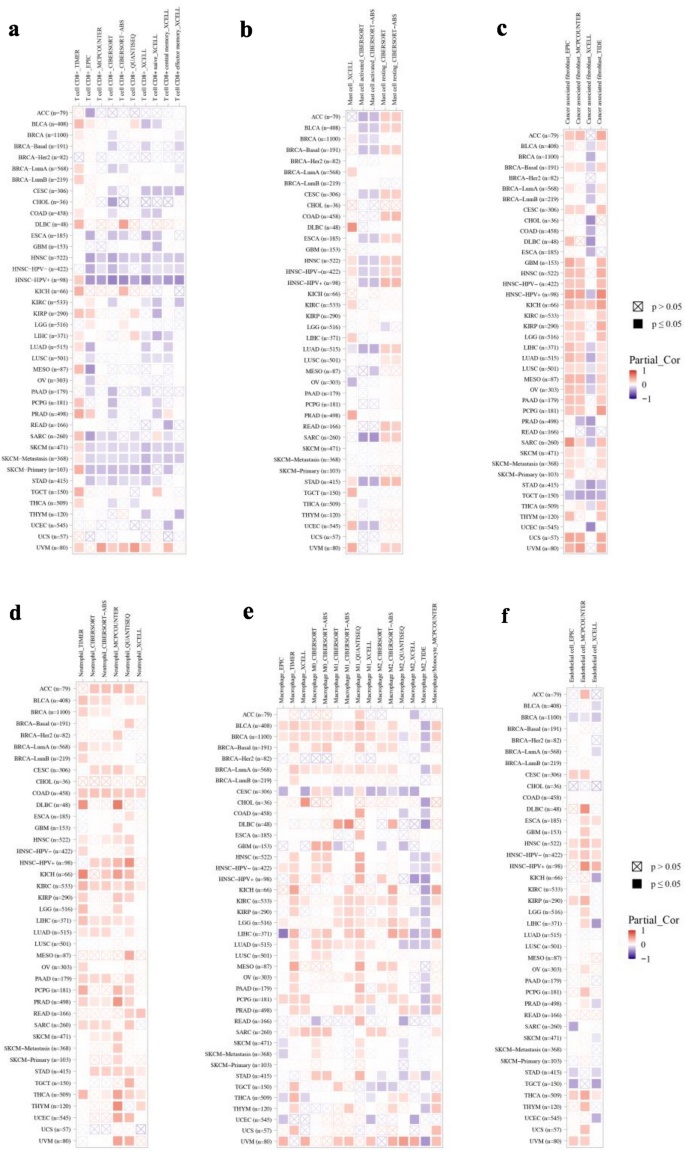
Pan-cancer analysis of LRRC59 and infiltration levels in different immune cells. a Correlations between LRRC59 and CD8+ T cells, b Correlations between LRRC59 and neutrophils, c Correlations between LRRC59 and macrophages, d Correlations between LRRC59 and mast cells, e Correlations between LRRC59 and cancer-associated fibroblasts and f Correlations between LRRC59 and endothelial cells
LRRC59 predicted the response to cancer immunotherapy
The results showed that the immune modulators ICGs and two predictive biomarkers for immunotherapy (TMB and MSI) had a close relationship with the expression levels of LRRC59 in pan-cancer. The majority of malignancies, including BLCA, KICH, KIRC, KIRP, LGG, LIHC, OV, PCPG, and UVM, exhibited a positive correlation between the expression levels of LRRC59 and the majority of ICGs. A few malignancies, such as CESC and SKCM, were inversely linked with the majority of ICGs (Fig. 7a). In addition, the expression levels of LRRC59 were favorably associated with TMB in patients with ACC, BLCA, BRCA, COAD, KIRC, LGG, LUAD, PAAD, SARC, SKCM, STAD and UCEC, whereas they were negatively associated with TMB in CESC and THCA patients. BLCA, KIRC, SARC, STAD, UCEC, and UVM displayed positive correlations with the LRRC59 expression, but DLBC, LGG, and PRAD displayed negative associations (Fig. 7b).
Fig. 7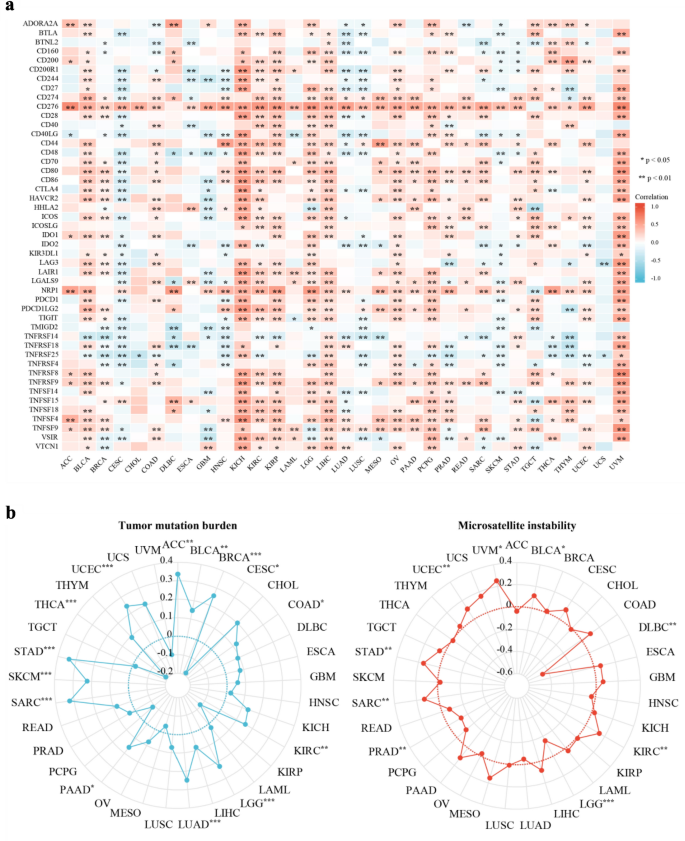
Correlations between LRRC59 expression and a ICGs, b TMB and MSI in pan-cancer. *p < 0.05, **p < 0.01, ***p < 0.001
LRRC59 was implicated in the regulation of several signaling pathways
Using gene set enrichment analysis (GSEA), we investigated the potential pathways through which LRRC59 may be involved in pan-cancer. According to the results of GSEA, the LRRC59 gene was related to the aberrant activation of 47 pathways. The most enriched signature pathways were related to endoplasmic reticulum homeostatic (the unfolded protein response and protein secretion), and cell proliferation (MYC targets V1, mTORC1 signaling, mitotic spindle, G2M checkpoint and E2F targets) (Fig. 8a) (Hetz et al., 2020; Schulze et al., 2020). The vast majority of hallmark pathways were enriched in a variety of cancers, particularly PAAD, UVM, PCPG, COAD and CHOL (Fig. 8b).
Fig. 8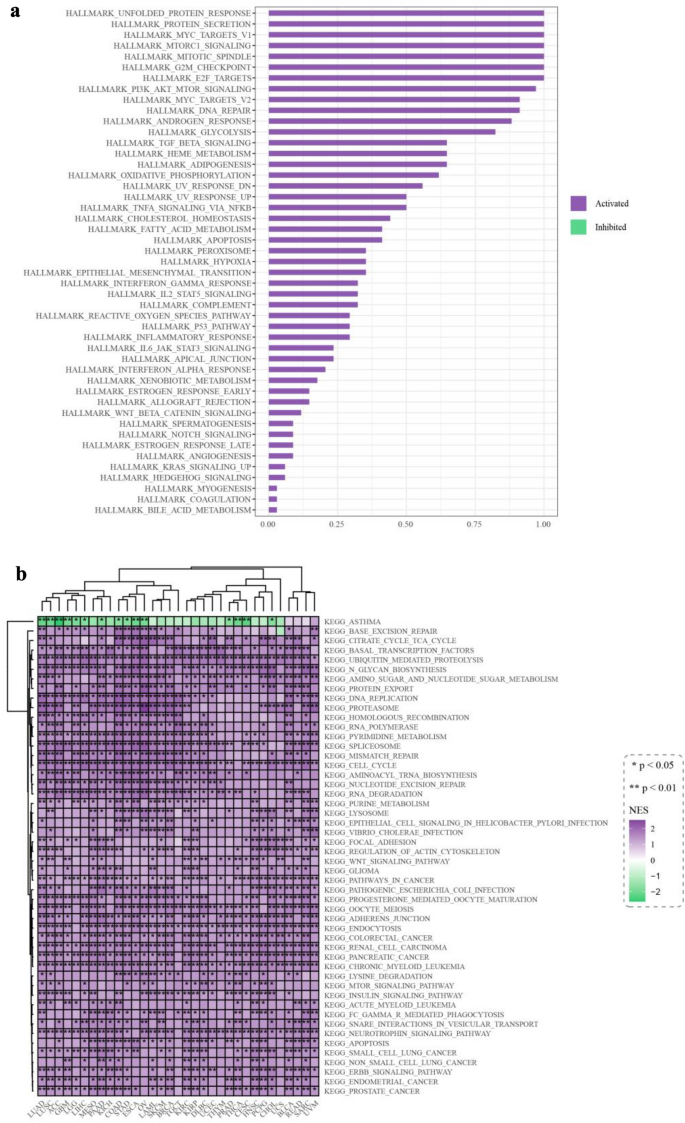
Gene set enrichment analysis of LRRC59 in pan-cancer. a Bar plot and b heatmap showing the results based on hallmark data set. *p < 0.05, **p < 0.01, ***p < 0.001
Genetic alterations of LRRC59 was relevant to survival prognosis
We performed a genome-wide pan-cancer analysis to assess the total number of mutations inside the LRRC59 gene. In 10,953 patients with different tumors, the results revealed 180 genetic variations of LRRC59, including mutation, structural variant, amplification, deep deletion and multiple alterations. The most prevalent type of LRRC59 alterations was copy number amplification, which was the only mutation type observed in tumor samples from MESO, ESCA, LIHC, PCPG, THYM and PRAD. Approximately 7.02% of UCS tumor samples exhibit the highest frequency of genetic alterations (Fig. 9a). In tumor samples, a total of 50 mutation sites were identified, representing 40 missense mutations, 3 truncating mutations, 3 splice mutations, and 4 structural variant and fusion variants (Fig. 9b). To estimate whether the genetic alterations of LRRC59 affected the survival prognosis, we constructed the survival curves, which indicated that altered LRRC59 showed better prognosis in PFS and OS than tumor samples without LRRC59 alteration, but DFS and DSS were not significantly different between the two groups (Fig. 9c).
Fig. 9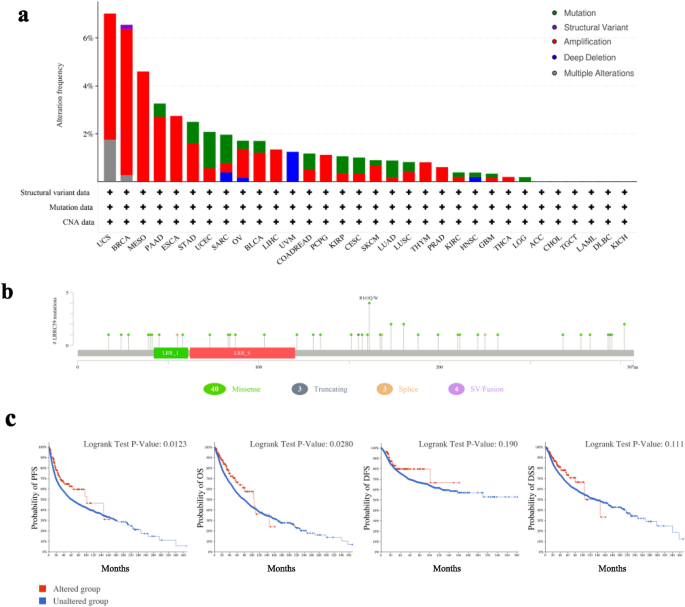
Analysis of LRRC59 gene alteration in pan-cancer based on cBioPortal database. a Alteration frequency with mutation type and b mutation site is displayed. c Correlations between LRRC59 gene alteration and progression-free survival and OS
LRRC59-related proteins are associated with tumorigenesis
We used STRING database to analyze those LRRC59-related proteins, and found FGF1 was one of them. FGF1 was found to be a key gene in the progression of cancer (Zhen et al., 2012), the authors proposed that LRRC59 facilitated the transportion of cytosolic FGF1 through nuclear pores by interacting with karyopherin and movement of LRRC59 along the endoplasmic reticulum and nuclear envelope membranes (Fig. 10).
Fig. 10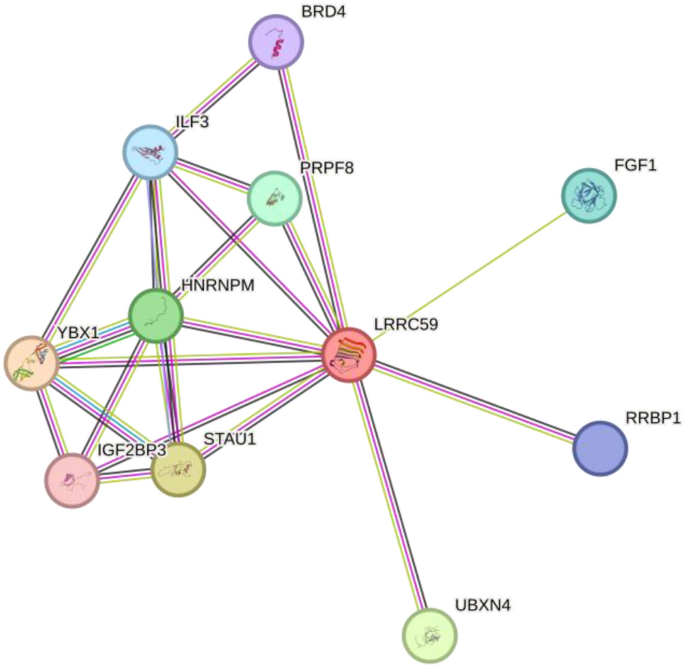
Protein–protein interaction between LRRC59 and other proteins, including BRD4, ILF3, PRPF8, HNRNPM, YBX1, IGF2BP3, STAU1, UBXN4, FGF1 and RRBP1
Discussion
LRRC59, a leucine-rich repeat-containing protein located at the endoplasmic reticulum (ER) interacting with ribosomes, was identified as a novel biomarker for urothelial carcinoma, breast cancer and lung cancer (Geng et al., 2021; Hannigan et al., 2020; Li et al., 2020; Pei et al., 2022; Toda et al., 2018). However, the mechanism of LRRC59 in the progression of other human malignancies has not been examined. Here, we conducted a comprehensive analysis of the expression pattern of LRRC59 across all malignancies in the TCGA database, along with its connection with clinicopathological staging, disease prognosis, gene mutations, immune cell infiltration. Our research revealed that LRRC59, a protein detected in the plasma, was a potential valuable biomarker for a variety of human malignancies. Our findings may provide new prospects for future clinical diagnosis and therapeutic targets.
The expression of LRRC59 at both mRNA and protein levels was significantly up-regulated in multiple cancer types. The protein expression of LRRC59 was revealed in 10 cancer types, among nine of them, mRNA and protein expression differences were consistent. Compared to normal samples, LRRC59 protein expression was down-regulated in PAAD tumor samples, while the LRRC59 mRNA expression was up-regulated, indicating other regulatory mechanisms. RNA degradation, protein degradation, post-translational modification and protein folding can also affect the gene expression resulting in the inconsistency between mRNA abundance and protein expression level.
LRRC59 was also a risk factor for patients of 11 types, including ACC, BLCA, CESC, HNSC, KICH, LGG, LIHC, LUAD, MESO, PAAD, and UVM, but a protective factor for COAD patients. The DFS analysis also confirmed that LRRC59 was a risk factor for patients with CESC and PAAD. The Kaplan–Meier survival curves indicated that increased LRRC59 expression was solely linked with better OS in patients with COAD while associating with worse OS in patients with ACC, BLCA, HNSC, KICH, KIRP, LGG, LIHC, LUAD, MESO, SKCM and UVM. All these data indicated LRRC59 was a valuable biomarker for cancer prognosis. The differential expression of LRRC59 and its correlation with various cancers probably implied the tissue specificity. Further tumor-type-specific studies needed to clarify the function of LRRC59 and particular tumor progression. Further analysis on plasma level of LRRC59 and specific tumor types will provide more information.
Gene mutation was commonly found in tumorigenesis. Copy-number variations were the most common type of somatic genetic events (Beroukhim et al., 2010) and the most prevalent kind of LRRC59 alteration in our analysis. Total of 50 mutations of LRRC59 were identified in tumor samples, including 40 missense mutations. The genetic alterations of LRRC59 were correlated with the better survival prognosis in PFS and OS. This indicated that LRRC59 was a valuable prognostic biomarker.
GSEA analysis showed that LRRC59 was involved in multiple pathways in pan-cancer, indicating a function link between LRRC59 and mRNA translation, protein trafficking and secretion in tumor progression. The LRRC59 gene correlated with aberrant activation of 47 pathways, including the unfolded protein response, protein secretion, MYC targets V1, mTORC1 signaling, mitotic spindle, G2M checkpoint and E2F targets, etc. Unfolded protein response, activated by accumulation of unfolded proteins in the ER, was associated with multiple diseases, including cancers (Chen & Cubillos-Ruiz, 2021; Hetz et al., 2020; Marciniak et al., 2022). Located at the ER membrane and nuclear envelope, LRRC59 was revealed to directly interact with mRNA translation factors and signal recognition particle machinery on the ER, therefore, regulating mRNA translation and protein secretion (Hannigan et al., 2020; Pei et al., 2022). A deeper understanding of the interaction of LRRC59 and these signaling pathways was needed. Besides, G2M Checkpoint, E2F Targets, MYC Targets V1, Mitotic Spindle were all key cell proliferation-related pathways. MYC, a well-known transcription factor, promoted cell growth and proliferation in multiple cancers (van Schaijik et al., 2018). MYC targets v1 was associated with breast cancer tumor aggressiveness and poor prognosis (Schulze et al., 2020). A crucial signaling in human cancer, mTORC1 signaling, also regulated cell growth, survival, autophagy and immunity (Hua et al., 2019). LRRC59 had been shown to promote cell proliferation in lung adenocarcinoma and urothelial carcinoma (Li et al., 2021; Pei et al., 2022). However, precise molecular mechanisms still needed to be explored.
LRRC59 contained leucine-rich repeat domains which were widely discovered in proteins involved in innate immunity and other cellular processes, such as apoptosis, autophagy and nuclear mRNA transport (Ng et al., 2011; Zhen et al., 2012). In fact, it had been demonstrated that LRRC59 mediated type I IFN signaling and antiviral immune responses (Xian et al., 2020). In our study, we displayed the consistent results among different algorithms from Timer2 database and discovered that LRRC59 expression was related to infiltrating immune cells in 33 cancer types in TCGA database. Particularly, there was a positive correlation between the expression levels of LRRC59 and the innate immunity markers neutrophils, total macrophage, M1 macrophages. The expression of LRRC59 was inversely associated with CD8+ T cell. These results show that LRRC59 modulated the interplay between innate and adaptive immunity in cancer by suppressing CD8+ T-cell function while simultaneously promoting neutrophil and macrophage function. Interestingly, the tumor malignancy-related endothelial cells were positively linked with LRRC59, which indicated LRRC59 may increase angiogenesis to support tumor growth and induce metastasis. However, more study is still required to fully understand the underlying mechanisms.
TMB was a predictive biomarker in solid tumors for the efficacy of immune checkpoint inhibitors (Sha et al., 2020). The expression of LRRC59 and ICGs/TMB/MSI across different tumors had been investigated. The results showed that the expression of LRRC59 correlated with the majority of ICGs and TMBs, indicating LRRC59 as a potential immune therapy target for most malignancies.
Overall, our findings showed that LRRC59 expression levels significantly differed among different types of human tumors and were correlated with the clinical characteristics and prognosis of pan-cancer patients. The expression of LRRC59 was tightly correlated with immune infiltration and TMB, and it may serve as a valuable biomarker and therapeutic target on prognosis in the majority of malignancies.
In conclusion, we conducted a comprehensive assessment of LRRC59, revealing its potential role as an indicator of patient prognosis and its immunoregulation effect.
Abbreviations
ACC: adrenocortical carcinoma; BLCA: bladder urothelial carcinoma; BRCA: breast invasive carcinoma; CESC: cervical squamous cell carcinoma and endocervical adenocarcinoma; CHOL: cholangiocarcinoma; COAD: colon adenocarcinoma; DLBC: Lymphoid neoplasm diffuse large B-cell lymphoma; ESCA: esophageal carcinoma; GBM: Glioblastoma multiforme; HNSC: head and neck squamous cell carcinoma; KICH: kidney chromophobe; KIRC: kidney renal clear cell carcinoma; KIRP: kidney renal papillary cell carcinoma; LAML: acute myeloid leukemia; LGG: brain lower grade glioma; LIHC: liver hepatocellular carcinoma; LUAD: lung adenocarcinoma; LUSC: lung squamous cell carcinoma; MESO: mesothelioma; OV: ovarian serous cystadenocarcinoma; PAAD: pancreatic adenocarcinoma; PCPG: pheochromocytoma and paraganglioma; PRAD: prostate adenocarcinoma; READ: rectum adenocarcinoma; SARC: sarcoma; SKCM: skin cutaneous melanoma; STAD: stomach adenocarcinoma; TGCT: testicular germ cell tumors; THCA: thyroid carcinoma; THYM: thymoma; UCEC: uterine corpus endometrial carcinoma; UCS: uterine carcinosarcoma; UVM: uveal melanoma.
Data availability
The data sets generated and/or analysed during the current study are available in the Gene Expression Profiling Interactive Analysis 2 (http://gepia2.cancer-pku.cn/#general, http://gepia2.cancer-pku.cn/#survival), Human Protein Atlas (https://www.proteinatlas.org/ENSG00000108829-LRRC59/blood+protein, https://www.proteinatlas.org/ENSG00000108829-LRRC59/subcellular), The Cancer Genome Atlas Program (https://portal.gdc.cancer.gov/repository?facetTab=files&filters=%7B%22op%22%3A%22and%22%2C%22content%22%3A%5B%7B%22op%22%3A%22in%22%2C%22content%22%3A%7B%22field%22%3A%22cases.project.program.name%22%2C%22value%22%3A%5B%22TCGA%22%5D%7D%7D%2C%7B%22op%22%3A%22in%22%2C%22content%22%3A%7B%22field%22%3A%22cases.project.project_id%22%2C%22value%22%3A%5B%22TCGA-ACC%22%2C%22TCGA-BLCA%22%2C%22TCGA-BRCA%22%2C%22TCGA-CESC%22%2C%22TCGA-CHOL%22%2C%22TCGA-COAD%22%2C%22TCGA-DLBC%22%2C%22TCGA-ESCA%22%2C%22TCGA-GBM%22%2C%22TCGA-HNSC%22%2C%22TCGA-KICH%22%2C%22TCGA-KIRC%22%2C%22TCGA-KIRP%22%2C%22TCGA-LAML%22%2C%22TCGA-LGG%22%2C%22TCGA-LIHC%22%2C%22TCGA-LUAD%22%2C%22TCGA-LUSC%22%2C%22TCGA-MESO%22%2C%22TCGA-OV%22%2C%22TCGA-PAAD%22%2C%22TCGA-PCPG%22%2C%22TCGA-PRAD%22%2C%22TCGA-READ%22%2C%22TCGA-SARC%22%2C%22TCGA-SKCM%22%2C%22TCGA-STAD%22%2C%22TCGA-TGCT%22%2C%22TCGA-THCA%22%2C%22TCGA-THYM%22%2C%22TCGA-UCEC%22%2C%22TCGA-UCS%22%2C%22TCGA-UVM%22%5D%7D%7D%2C%7B%22op%22%3A%22in%22%2C%22content%22%3A%7B%22field%22%3A%22files.analysis.workflow_type%22%2C%22value%22%3A%5B%22STAR%20-%20Counts%22%5D%7D%7D%2C%7B%22op%22%3A%22in%22%2C%22content%22%3A%7B%22field%22%3A%22files.data_category%22%2C%22value%22%3A%5B%22transcriptome%20profiling%22%5D%7D%7D%2C%7B%22op%22%3A%22in%22%2C%22content%22%3A%7B%22field%22%3A%22files.experimental_strategy%22%2C%22value%22%3A%5B%22RNA-Seq%22%5D%7D%7D%5D%7D, https://portal.gdc.cancer.gov/repository?facetTab=cases&filters=%7B%22op%22%3A%22and%22%2C%22content%22%3A%5B%7B%22op%22%3A%22in%22%2C%22content%22%3A%7B%22field%22%3A%22cases.project.program.name%22%2C%22value%22%3A%5B%22TCGA%22%5D%7D%7D%2C%7B%22op%22%3A%22in%22%2C%22content%22%3A%7B%22field%22%3A%22cases.project.project_id%22%2C%22value%22%3A%5B%22TCGA-ACC%22%2C%22TCGA-BLCA%22%2C%22TCGA-BRCA%22%2C%22TCGA-CESC%22%2C%22TCGA-CHOL%22%2C%22TCGA-COAD%22%2C%22TCGA-DLBC%22%2C%22TCGA-ESCA%22%2C%22TCGA-GBM%22%2C%22TCGA-HNSC%22%2C%22TCGA-KICH%22%2C%22TCGA-KIRC%22%2C%22TCGA-KIRP%22%2C%22TCGA-LAML%22%2C%22TCGA-LGG%22%2C%22TCGA-LIHC%22%2C%22TCGA-LUAD%22%2C%22TCGA-LUSC%22%2C%22TCGA-MESO%22%2C%22TCGA-OV%22%2C%22TCGA-PAAD%22%2C%22TCGA-PCPG%22%2C%22TCGA-PRAD%22%2C%22TCGA-READ%22%2C%22TCGA-SARC%22%2C%22TCGA-SKCM%22%2C%22TCGA-STAD%22%2C%22TCGA-TGCT%22%2C%22TCGA-THCA%22%2C%22TCGA-THYM%22%2C%22TCGA-UCEC%22%2C%22TCGA-UCS%22%2C%22TCGA-UVM%22%5D%7D%7D%2C%7B%22op%22%3A%22in%22%2C%22content%22%3A%7B%22field%22%3A%22files.data_category%22%2C%22value%22%3A%5B%22clinical%22%5D%7D%7D%2C%7B%22op%22%3A%22in%22%2C%22content%22%3A%7B%22field%22%3A%22files.data_format%22%2C%22value%22%3A%5B%22bcr%20xml%22%5D%7D%7D%5D%7D), UALCAN (https://ualcan.path.uab.edu/cgi-bin/Pan-cancer-CPTAC.pl?genenam=LRRC59), Tumor Immune Estimation Resource version 2 (http://timer.comp-genomics.org), GTBAdb panGTBA analysis (http://guotosky.vip:13838/GTBA/), cBioPortal (http://www.cbioportal.org/results/cancerTypesSummary?case_set_id=all&gene_list=LRRC59&cancer_study_list=5c8a7d55e4b046111fee2296, http://www.cbioportal.org/results/mutations?case_set_id=all&gene_list=LRRC59&cancer_study_list=5c8a7d55e4b046111fee2296, http://www.cbioportal.org/results/comparison?case_set_id=all&gene_list=LRRC59&cancer_study_list=5c8a7d55e4b046111fee2296&comparison_subtab=survival) and [S-BSST1079] repository, [https://www.ebi.ac.uk/biostudies/studies/S-BSST1079?query=S-BSST1079]. The R code is released with an opensource license and may be accessed at: [LRRC59] repository, [https://github.com/Tsangmeikei/LRRC59].
References
Beroukhim, R., Mermel, C. H., Porter, D., Wei, G., Raychaudhuri, S., Donovan, J., Barretina, J., Boehm, J. S., Dobson, J., Urashima, M., Mc Henry, K. T., Pinchback, R. M., Ligon, A. H., Cho, Y.-J., Haery, L., Greulich, H., Reich, M., Winckler, W., Lawrence, M. S., et al. (2010). The landscape of somatic copy-number alteration across human cancers. Nature, 463(7283), 899–905. https://doi.org/10.1038/nature08822.
Chen, F., Chandrashekar, D. S., Varambally, S., & Creighton, C. J. (2019). Pan-cancer molecular subtypes revealed by mass-spectrometry-based proteomic characterization of more than 500 human cancers. Nature Communications, 10(1), 5679. https://doi.org/10.1038/s41467-019-13528-0.
Chen, X., & Cubillos-Ruiz, J. R. (2021). Endoplasmic reticulum stress signals in the tumour and its microenvironment. Nature Reviews. Cancer, 21(2), 71–88. https://doi.org/10.1038/s41568-020-00312-2.
Geng, X., Zhang, Y., Zeng, Z., Zhu, Z., Wang, H., Yu, W., & Li, Q. (2021). Molecular characteristics, prognostic value, and immune characteristics of m6A regulators identified in head and neck squamous cell carcinoma. Frontiers in Oncology, 11, 629718. https://doi.org/10.3389/fonc.2021.629718.
Hannigan, M. M., Hoffman, A. M., Thompson, J. W., Zheng, T., & Nicchitta, C. V. (2020). Quantitative proteomics links the LRRC59 interactome to mRNA translation on the ER membrane. Molecular & Cellular Proteomics: MCP, 19(11), 1826–1849. https://doi.org/10.1074/mcp.RA120.002228.
Hetz, C., Zhang, K., & Kaufman, R. J. (2020). Mechanisms, regulation and functions of the unfolded protein response. Nature Reviews. Molecular Cell Biology, 21(8), 421–438. https://doi.org/10.1038/s41580-020-0250-z.
Hua, H., Kong, Q., Zhang, H., Wang, J., Luo, T., & Jiang, Y. (2019). Targeting mTOR for cancer therapy. Journal of Hematology & Oncology, 12(1), 71. https://doi.org/10.1186/s13045-019-0754-1.
Li, D., Xing, Y., Tian, T., Guo, Y., & Qian, J. (2020). Overexpression of LRRC59 is associated with poor prognosis and promotes cell proliferation and invasion in lung adenocarcinoma. OncoTargets and Therapy, 13, 6453–6463. https://doi.org/10.2147/OTT.S245336.
Li, S., Dong, C., Chen, J., Gao, X., Xie, X., & Zhang, X. (2021). Identification of an immune checkpoint gene signature that accurately predicts prognosis and immunotherapy response in endometrial carcinoma. Aging, 13(12), 16696–16712. https://doi.org/10.18632/aging.203189.
Marciniak, S. J., Chambers, J. E., & Ron, D. (2022). Pharmacological targeting of endoplasmic reticulum stress in disease. Nature Reviews. Drug Discovery, 21(2), 115–140. https://doi.org/10.1038/s41573-021-00320-3.
Maurizio, E., Wiśniewski, J. R., Ciani, Y., Amato, A., Arnoldo, L., Penzo, C., Pegoraro, S., Giancotti, V., Zambelli, A., Piazza, S., Manfioletti, G., & Sgarra, R. (2016). Translating proteomic into functional data: An High Mobility Group A1 (HMGA1) proteomic signature has prognostic value in breast cancer. Molecular & Cellular Proteomics: MCP, 15(1), 109–123. https://doi.org/10.1074/mcp.M115.050401.
Ng, A. C. Y., Eisenberg, J. M., Heath, R. J. W., Huett, A., Robinson, C. M., Nau, G. J., & Xavier, R. J. (2011). Human leucine-rich repeat proteins: A genome-wide bioinformatic categorization and functional analysis in innate immunity. Proceedings of the National Academy of Sciences of the United States of America, 108(Suppl 1), 4631–4638. https://doi.org/10.1073/pnas.1000093107.
Pallai, R., Bhaskar, A., Barnett-Bernodat, N., Gallo-Ebert, C., Pusey, M., Nickels, J. T., & Rice, L. M. (2015). Leucine-rich repeat-containing protein 59 mediates nuclear import of cancerous inhibitor of PP2A in prostate cancer cells. Tumour Biology: THe Journal of the International Society for Oncodevelopmental Biology and Medicine, 36(8), 6383–6390. https://doi.org/10.1007/s13277-015-3326-1.
Pei, L., Zhu, Q., Zhuang, X., Ruan, H., Zhao, Z., Qin, H., & Lin, Q. (2022). Identification of leucine-rich repeat-containing protein 59 (LRRC59) located in the endoplasmic reticulum as a novel prognostic factor for urothelial carcinoma. Translational Oncology, 23, 101474. https://doi.org/10.1016/j.tranon.2022.101474.
Schulze, A., Oshi, M., Endo, I., & Takabe, K. (2020). MYC targets scores are associated with cancer aggressiveness and poor survival in ER-positive primary and metastatic breast cancer. International Journal of Molecular Sciences, 21(21), 8127. https://doi.org/10.3390/ijms21218127.
Sha, D., Jin, Z., Budczies, J., Kluck, K., Stenzinger, A., & Sinicrope, F. A. (2020). Tumor mutational burden as a predictive biomarker in solid tumors. Cancer Discovery, 10(12), 1808–1825. https://doi.org/10.1158/2159-8290.CD-20-0522.
Siegel, R. L., Miller, K. D., Fuchs, H. E., & Jemal, A. (2022). Cancer statistics, 2022. CA: A Cancer Journal for Clinicians, 72(1), 7–33. https://doi.org/10.3322/caac.21708.
Tatematsu, M., Funami, K., Ishii, N., Seya, T., Obuse, C., & Matsumoto, M. (2015). LRRC59 regulates trafficking of nucleic acid-sensing TLRs from the endoplasmic reticulum via association with UNC93B1. Journal of Immunology (baltimore, Md.: 1950), 195(10), 4933–4942. https://doi.org/10.4049/jimmunol.1501305.
Toda, H., Kurozumi, S., Kijima, Y., Idichi, T., Shinden, Y., Yamada, Y., Arai, T., Maemura, K., Fujii, T., Horiguchi, J., Natsugoe, S., & Seki, N. (2018). Molecular pathogenesis of triple-negative breast cancer based on microRNA expression signatures: Antitumor miR-204-5p targets AP1S3. Journal of Human Genetics, 63(12), 1197–1210. https://doi.org/10.1038/s10038-018-0510-3.
van Schaijik, B., Davis, P. F., Wickremesekera, A. C., Tan, S. T., & Itinteang, T. (2018). Subcellular localisation of the stem cell markers OCT4, SOX2, NANOG, KLF4 and c-MYC in cancer: A review. Journal of Clinical Pathology, 71(1), 88–91. https://doi.org/10.1136/jclinpath-2017-204815.
Vivian, J., Rao, A. A., Nothaft, F. A., Ketchum, C., Armstrong, J., Novak, A., Pfeil, J., Narkizian, J., Deran, A. D., Musselman-Brown, A., Schmidt, H., Amstutz, P., Craft, B., Goldman, M., Rosenbloom, K., Cline, M., O’Connor, B., Hanna, M., Birger, C., et al. (2017). Toil enables reproducible, open source, big biomedical data analyses. Nature Biotechnology, 35(4), 314–316. https://doi.org/10.1038/nbt.3772.
Xian, H., Yang, S., Jin, S., Zhang, Y., & Cui, J. (2020). LRRC59 modulates type I interferon signaling by restraining the SQSTM1/p62-mediated autophagic degradation of pattern recognition receptor DDX58/RIG-I. Autophagy, 16(3), 408–418. https://doi.org/10.1080/15548627.2019.1615303.
Yu, Y. P., Ding, Y., Chen, Z., Liu, S., Michalopoulos, A., Chen, R., Gulzar, Z. G., Yang, B., Cieply, K. M., Luvison, A., Ren, B.-G., Brooks, J. D., Jarrard, D., Nelson, J. B., Michalopoulos, G. K., Tseng, G. C., & Luo, J.-H. (2014). Novel fusion transcripts associate with progressive prostate cancer. The American Journal of Pathology, 184(10), 2840–2849. https://doi.org/10.1016/j.ajpath.2014.06.025.
Zhen, Y., Sørensen, V., Skjerpen, C. S., Haugsten, E. M., Jin, Y., Wälchli, S., Olsnes, S., & Wiedlocha, A. (2012). Nuclear import of exogenous FGF1 requires the ER-protein LRRC59 and the importins Kpnα1 and Kpnβ1. Traffic (copenhagen, Denmark), 13(5), 650–664. https://doi.org/10.1111/j.1600-0854.2012.01341.x.
Funding
This research was supported by the National Natural Science Foundation of China (No. 81773939) and the Science and Technology Planning Fundamental Research Project of Shenzhen (No. JCYJ20210324094005015).
Author information
Meiqi Zeng and Xia Wang have contributed equally to this work.
Authors and Affiliations
Marshall Laboratory of Biomedical Engineering, Shenzhen University, Shenzhen, China
Meiqi Zeng, Zhenguang Ying & Ou Sha
School of Dentistry, Shenzhen University Medical School, Shenzhen University, Shenzhen, China
Meiqi Zeng, Zhenguang Ying, Feng Gao, Xianxiong Chen & Ou Sha
Department of Anatomy and Histology, School of Basic Medical Sciences, Shenzhen University Medical School, Shenzhen University, Shenzhen, China
Xia Wang, Xiaona Wang, Yuning Zhang & Ou Sha
Department of Biochemistry and Molecular Biology, School of Basic Medical Sciences, Shenzhen University Medical School, Shenzhen University, Shenzhen, China
Lixin Xia
Faculty of Health Sciences, University of Macau, Macau, China
Kin Yip Tam
Department of Gastroenterology and Hepatology, Shenzhen University General Hospital, Shenzhen, China
Long Xu
Shenzhen University Clinical Medical Academy, Shenzhen, China
Long Xu
International Cancer Center, Shenzhen University Medical School, Shenzhen University, Shenzhen, China
Long Xu
Contributions
Conceptualization, MZ and OS; formal analysis, XW, YZ and ZY; writing—original draft, MZ and XW; writing—review and editing, LX, FG, XC, KYT, LX and OS.
Corresponding authors
Ethics declarations
Conflict of interest
The authors declare that the research was conducted in the absence of any commercial or financial relationships that could be construed as a potential conflict of interest. The authors declare that they have no competing interests.
Rights and permissions
Springer Nature or its licensor (e.g. a society or other partner) holds exclusive rights to this article under a publishing agreement with the author(s) or other rightsholder(s); author self-archiving of the accepted manuscript version of this article is solely governed by the terms of such publishing agreement and applicable law.
About this article
Cite this article
Zeng, M., Wang, X., Wang, X. et al. An integrated pan-cancer analysis of leucine-rich repeat containing protein 59: a potential biomarker for prognostic and immunotherapy. GENOME INSTAB. DIS. 4, 333–348 (2023). https://doi.org/10.1007/s42764-023-00113-5
Received20 July 2023
Revised06 September 2023
Accepted20 September 2023
Published21 November 2023
Issue DateDecember 2023
DOIhttps://doi.org/10.1007/s42764-023-00113-5
Share this article
Anyone you share the following link with will be able to read this content:
Get shareable link
用户登录
还没有账号?
立即注册