MiR-146a induces the aging of mesenchymal stem cell via CASK
Original Research Paper
Published: 17 January 2024
Yanxia Zhu, Jia Liu, Yiyang Liu, Junyu Su, Kangling Xu & Guangqian Zhou
Genome Instability & Disease Volume 5, pages 35–44, (2024)
Abstract
Mesenchymal stem cell (MSC) has elicited great hopes for regenerative medicine, however, its regenerative potential decreases with age, which raises questions about the efficiency of autologous transplantations in elderly patients. To elucidate the cellular consequences and epigenetic mechanisms of aging, the functional and epigenetic changes in bone marrow derived MSC (BMSC) from normal and aging rats were studied concurrently. The results demonstrate that the proliferation and differentiation abilities of BMSCs from aging rats declined compared with those from young rats. In order to identify which miRNA involve in the senescence of BMSC, miRNA expression profiles of both BMSCs from young and aging rats were examined. The results showed that miR-146a expression increased significantly in BMSCs from aging rats compared with that from young rats, following with a decrease in Calcium/calmodulin-dependent Serine protein kinase (CASK) expression. Inhibition of miR-146a can reduces the numbers of senescent cells and stimulates the differentiation of BMSC. Luciferase assay demonstrated that CASK was a direct target of miR-146a, inhibition of miR-146a significantly elevated the expression of CASK. Overexpression of CASK in aged BMSC can rescue its proliferation and differentiation abilities. All these results indicate that miR-146a may cause aging and senescence of BMSC through the inhibition of CASK expression, which may be potential target for BMSC aging and some age-related disease.
Introduction
Mesenchymal stem cells (MSCs) have great potential for application in cell-based therapies and tissue engineering approaches (Liu et al., 2022), because of their potential for self-renewal and multilineage differentiation. However, the decline in the regenerative capacity of resident stem cells across different tissues is probably one of the main reasons for whole body aging, animal and clinical studies also proved that the regeneration potential of many tissues declines with age (Yamakawa et al., 2020). Consequently, treatment with MSCs from older donors appears to be less effective than application of their younger counterparts (Bustos et al., 2014; Kasper et al., 2009). If the intrinsic changes in MSCs caused age-dependent decrease in regenerative potential, autologous transplantation are prone to be suboptimal in elderly patients, who have the most need for such therapies. Understanding the underlying mechanism leading to stem cell aging can help us improving tissue maintenance and function during aging, thus reducing the suffering of age-related diseases.
The molecular basis of human aging is being investigated in many experiments, including telomere shortening, DNA damage and oxidative stress (Cai et al., 2022; López-Otín et al., 2023) as well as changes in gene expression. Researchers also proposed that epigenetic factors, such as microRNAs (miRNAs), may also be important to control gene expression changes and genomic instability during aging (Duan et al., 2022). Although the contribution of epigenetics to several human diseases has been proved (Cavalli et al., 2019; Zhang et al., 2020), the epigenetic variations of BMSC owing to aging remain poorly understood.
It has been implicated that miRNA regulate the aging and cellular senescence. miRNA deregulation is an emerging and promising field in age-related epigenetics. Many studies have shown that the change of miRNA levels during the species lifespan can be associated with age-related disorders (Demirel-Yalciner et al., 2022). A miRNA expression array performed in the mice livers showed more upregulated than downregulated miRNAs during aging (Ghafouri-Fard et al., 2021). In the nematode, knocking out the miRNA gene lin-4 decreased the lifespan and accelerated tissue aging, while overexpression of this miRNA extended it (Boehm et al., 2005), this effect was mediated by insulin-IGF1 pathway which closely involved with aging in many different organisms. A correlation between four miRNAs (miR-93, miR-669c, miR-214, and miR-709) and expression of the corresponding gene targets associated with mitochondrial function, oxidative stress, and proliferation were demonstrated by proteomic and miRNA profiling, which indicates that these miRNAs are relate with aging. Some other aging associated miRNAs such as miR-143, miR-27 and miR-29, is rapidly increasing in all kinds of aging models (Bonifacio et al., 2010). In rheumatoid arthritis (RA) patients, the expression of miR-146a is stimulated by inflammatory cytokines (Zhang et al., 2023), which means miR-146a may be related with aging diseases.
To date, no profiling studies have been performed in bone marrow mesenchymal stem cell from aging rats, and little is known about whether or how miRNA targets any protein to regulate the aging of BMSC. In this work, we have evaluated the miRNA expression profile of BMSC from aging rat for the first time. We report that the expression of the miR-146a is upregulated in aging rat BMSC. In addition, we have found that miR-146a bind to the 3’-UTR of CASK directly as a natural inhibitor to regulate BMSCs senescence, inhibition of miR-146a activity can rescue cellular senescence and increase differentiation potential of BMSC. Therefore, miR-146a and CASK may be potential target for the therapy of age-related diseases.
Materials and methods
Animals and treatment
Six to eight-week-old Wistar rats (180–200 g) were randomly divided into two groups of 15 each. After 1 week adaptation period, the animals were given one of the following preparations subcutaneously for 8 weeks: (I) 0.4 mL PBS as vehicle control; (II) D-galactose at 120 mg/kg by intragastric injection daily for 8 weeks. Rats were sacrificed at the end of treatment and bone marrow was collected immediately for the isolation of BMSCs; sera and tissues were immediately collected for experiments or stored at – 80 °C for later examinations. All experimental procedures used in this study had been approved by the ethics committee in this institute and all animal experiments had been performed in accordance with the ethical standards laid down in the 1964 Declaration of Helsinki. The authors who performed experiments had given their informed consent prior to the study and had followed “principles of laboratory animal care”.
SOD, GSH-Px and MDA measurement
To detect the oxidative stress level, we examined the total SOD activity, GSH-Px activity and MDA content, Rats’ sera were collected after sacrificing. SOD activity was measured using the xanthine oxidase method, GSH-Px activity was examined with 5, 5′-dithiobis (2-nitrobenzoic acid) (DTNB), while the MDA content was estimated with the thiobarbituric acid (TBA) method following the manufacturer’s instructions (Jiancheng Institute, Nanjing, China).
BMSC culture
Bone marrow was harvested from control and treated rats separately. The femurs and tibias were removed from the rats and bone marrow was flushed out of the bones using 10 ml PBS with 100 U/ml heparin in a syringe. The cells were centrifuged at 1000 rpm for 8 min. The cell pellet was resuspended in 10 mL Dulbecco’s modified Eagle’s medium (DMEM; Gibco, USA) supplemented with 10% fetal bovine serum (FBS, Gibco, USA) and plated in a 25-cm2 plastic flask to allow the MSCs to adhere. The medium was completely replaced every 3 days. All the experiments described below were performed with BMSCs after the fourth passage.
SA-β-gal staining
Senescence-associated β-galactosidase (SA-β-gal) staining was performed using a SA-β-gal staining kit (Genmed Scientifics Inc., China) following the manufacturer’s protocol. BMSCs at passage 5 were used for the staining. The cells were fixed in 4% (v/v) formaldehyde for 5 min and were stained with SA-β-gal staining solution at pH 6.0 for 12 h. The SA-β-gal-positive cells exhibited as blue color.
Proliferation and differentiation assays
Cultured cells in single-cell suspension were counted using a hemocytometer, and 60 cells were plated in each 10-cm culture dish for the colony-forming unit (CFU) assay. After 2 weeks, colonies were fixed with 100% methanol, stained with crystal violet, and counted.
The proliferation ability was analyzed by cell counting kit (cck-8). BMSCs of passage 5 with 5 × 103 cells /ml were plated in 96-well plate to develop growth curves. 10 µl of sterile cck-8 were added to each well and incubated for 3 h at 37 °C. The percentage of viable cells was determined every day for 2 weeks. The optical density values were determined at least in triplicate against a reagent blank at a test wavelength of 450 nm and reference wavelength of 630 nm.
BMSCs at passage 5 were differentiated into adipocytes, osteoblasts and chondrocytes in corresponding induction medium as previously described (Zhu et al., 2008). Then stained with Oil Red-O to detect lipid; stained with Alzarin Red for calcium deposition, stained with Toluidine Blue for chondrogenic GAGs. After differentiation, total RNA from each group was also extracted, real time-PCR were performed to detect RNA expression using the Power SYBR® Green PCR Master Mix (Takara, China) according to the manufacturer’s protocol.
miRNA expression profiling
Total RNA was extracted from BMSCs with TRIzol (Invitrogen). About 200 ng of RNA was used for the hybridization procedure. The miRNAs were ligated to a biotinylated signal molecule using the FlashTag Biotin HSR RNA Labeling Kit (Genisphere, Hatfield, PA, USA). The Affymetrix miRNA arrays were scanned on a GeneChip Scanner 3000 7G System (Affymetrix). Robust multichip average expression values were obtained and tested for differential gene expression using Welch’s two-sample t test assuming unequal variances, at a P < 0.05 threshold. The principle component analysis (PCA) is run as an R-Analysis in which the variables (p) are put in a pxp dispersion matrix (covariance) of variables where each transformed observation is represented by its principle components.
mRNA expression profiling
Total RNA was extracted, and RNA integrity was determined using agarose gel electrophoresis. Target preparation was done for individual samples, and hybridization to RAE230A arrays, staining, washing and scanning of the chips were performed according to the manufacturer’s technical manual (Affymetrix). The Microarray Analysis Suite 5.0 (MAS5) produced cell intensity files that were inspected for regional hybridization bias and quality control parameters as described. The MAS5 recognized 60% of the 15,800 probe sets on the RAE230A array as present in our samples. CEL files were imported into the Partek Genomics Suite. The data were log2 normalized. RNA expression values were obtained and tested for differential gene expression using Welch’s two-sample t test assuming unequal variances, at a P < 0.05 threshold.
Quantitative RT-PCR analysis
S-Poly(T) miRNA qPCR-assay (mirnalab, China) was used to detect the expression of miRNA146. Total RNA from cultured cells was polyadenylated and reverse transcriptase with PolyA/RT enzyme mix. The RT products can be amplified and detected using a universal Taqman probe. The miR146 expression level was normalized to the expression level of snoRNA-202 and calculated using the method.
Western blot analysis
BMSCs were lysed in RIPA buffer (Thermo Fisher, USA). A 40 μg sample of the total protein was resolved using SDS-PAGE and transferred onto polyvinylidene difluoride (PVDF, Millipore, USA) membranes. The membranes were blocked with 5% non-fat milk. Primary antibodies to detect CASK (1:1000, Sigma-Aldrich, USA) and β-actin (1:1000, Sigma-Aldrich, USA) were incubated overnight with the membranes at 4 °C. Membranes were incubated with horseradish peroxidase (HRP)-conjugated secondary antibodies, and proteins were detected by enhanced chemiluminescence (ECL) (Amersham Biosciences Corp, USA). β-actin was used as the internal control to normalize the loading materials.
Luciferase assays
The luciferase reporter was constructed based on the pMIR-REPORT vector (Ambion, Austin, TX, USA). The sense and anti-sense strands of the oligonucleotides of the CASK-3’-UTR containing the miR-146a-binding site were synthesized, annealed, and then subcloned into the pMIR-REPORT vector. The scrambled sequences were subcloned into the same vector in order to act as negative control. The CASK-3′-UTR-miR146a or control plasmid was transfected into the HEK293 stable cell line using Lipofectamine 2000 (Invitrogen, Eugene, OR, USA). phRL-TK Renilla luciferase plasmid (Promega, Madison, WI, USA) was cotransfected for normalization of transfection efficiency. Both Renilla and Firefly luciferase activity of each sample were measured in a TD-20/20 luminometer (Turner Biosystems).
Cell transfections
Transfection of BMSCs was carried out using Entranster™ (Engreen, China) following manufacturer’s instructions. BMSCs were seeded in 24-well plates and the miRNA precursor or inhibitory molecules (from Shanghai Integrated Biotech Solutions Co., Ltd) were transfected at 0.67 µg per 24-well plate. miRNA inhibition was carried out using a control or miR-146a inhibitor (IBS, Shanghai). miRNA over-expression in BMSCs was performed using miR-146a mimic or control precursor molecules (IBS, Shanghai).
Inducible overexpression of CASK and small interfering RNA to CASK
The full-length cDNA of CASK was amplified by PCR, and inserted in the pCDNA3.1 plasmids with EcoRI and XbaI sites. The plasmids were transfected into BMSCs using the Lipofectamine 2000 reagent (Invitrogen).
RNAi was undertaken using the pBS/U6 vector (IBS, Shanghai) to direct expression of the relevant hairpin double-stranded sequence. A sequence of 19 nucleotides within the hCASK coding region immediately downstream of AA dinucleotides was chosen, which locates + 152 to + 170 base pair downstream of AUG start codon. Two oligonucleotides, 5′-G T C C A G G G T T A AGTACAGATTCAGATCTGTACTTAACCCTGGACTTTTTT-3′ and 5′-AATTAAAAAAGTCCAGGGT TAAGTACAGATCTGAATCTGTACTTAACCCT G G A C G G C C-3′, were synthesized, annealed, and cloned into pBS/U6 vectors between the ApaI and EcoRI sites. Sequencing validated the positive reconstructs. BMSCs were transfected with expression plasmids of CASK siRNA or empty vector pBS/U6 by FuGENE6 reagent (Roche) according to the manufacturer’s instructions.
Statistical analyses
Each experiment was repeated at least three times. Data are presented as means ± SD. Statistical analysis of the data was performed using a standard two-sample Student’s t test. Statistical significance was determined using a two-tailed distribution assumption. Asterisks and pound represent a significant difference between values of experimental pairs with P < 0.05.
Results
Aging BMSCs showed susceptible senescence and loss of stemness
To identify the aging of D-galactose induced rats, Rats’ sera were collected and oxidative biomarkers were examined. Rats treated with D-galactose did not show obvious abnormality (e.g., feed intake, water intake, body weight) (data not shown). Results showed that D-gal-administration caused an increase in MDA level and a decrease in T-SOD and GSH-Px activities in serum (Fig. 1A–C). To identify the aging of BMSC from D-galactose treated rat, β-galactosidase staining showed that the cultured BMSCs showed more β-galactosidase positive cells than that of BMSCs from normal rats (Fig. 1D). The total number of CFUs was significantly decreased compared with that of BMSC from normal rats. Similarly, the number of alkaline phosphatase -positive CFUs was reduced in D-galactose treated rat (Fig. 1E). Cell counting kit-8 were used to examine the proliferation ability of BMSC, results showed that BMSC from D-galactose treated rat have lower cellular proliferation rate than that of BMSC from young rats (Fig. 1F). To determine the role of D-galactose on the differentiation capacity of BMSCs in inducing aging, BMSCs were differentiated into osteogenic, chondrogenic and adipogenic lineages, and the results showed that the differentiation potency of BMSCs to all three lineages was decreased in the artificial aging group compared with the control group (Fig. 1G). Furthermore, we detected mRNA levels of osteogenic marker genes (OPN, OGC, Col1, RUNX-2), adipogenic marker genes (PPAR-γ, adiponectin), and chondrogenic marker genes (Col2a1 and Sox9) in BMSCs and found that the expression of these marker genes was decreased in the aging rats compared with that of young rats (Fig. 1G, H).
Fig. 1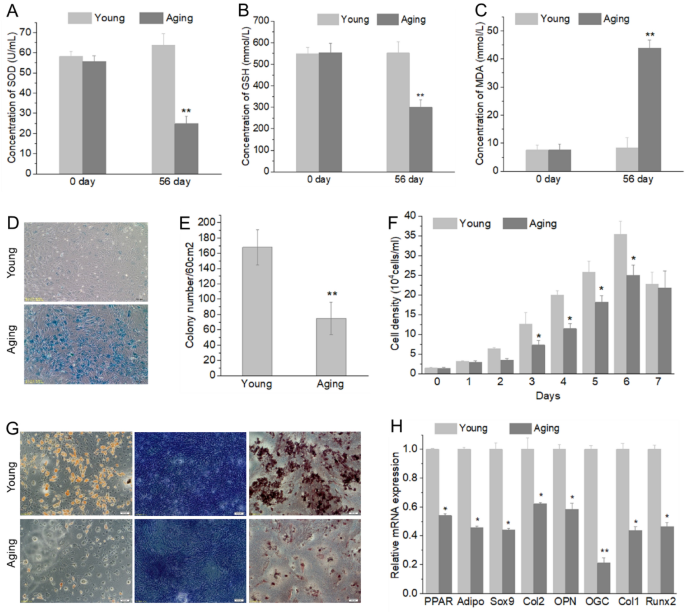
The proliferation and differentiation ability of BMSCs from young and aging rats. Changes of SOD (A), GSH-PX activity (B) and MDA content (C) in ageing rats were induced by D-galactose; Senescent cells showed blue after stained with ß-galactosidase (D); Percentage of AP-positive CFUs in aging BMSCs was decreased significantly (E), CFUs were considered as AP-positive when more than 50% of cells stained AP; BMSC from D-galactose treated rat have lower proliferation (F) and differentiation (G, H) ability than that of BMSC from normal rats. Adipogenic differentiation determined by lipid droplet (Oil Red staining), chondrogenic differentiation determined by GAGs (Toluidine Blue staining), osteogenic differentiation determined by matrix mineralization (Alizarin Red staining). Five independent experiments were carried out for all assays. *P < 0.05, **P < 0.01, compare with Young group. SOD superoxide dismutase, GSH-PX glutathione peroxidase, MDA malondialdehyde, AP alkaline phosphatase, CFU colony-forming unit, Young young cells, Aging aged cells
miR-146a is involved in the BMSC aging and loss of stemness
To investigate which miRNA and pathway may involve in the aging process of BMSC, we analyze miRNA expression of aging and young BMSCs, 1367 miRNAs were profiled, among them, 85 miRNAs were up-regulated, while 61 miRNAs were down-regulated (Fig. 2A). Among the up-regulated miRNAs, miRNA146a was the highest up-regulated miRNA (Fig. 2B). The expression level of miR146a was also validated by real-time PCR, which further confirmed the up-regulation of miR146a in BMSCs from D-gal-induced aging rats (Fig. 2C).
Fig. 2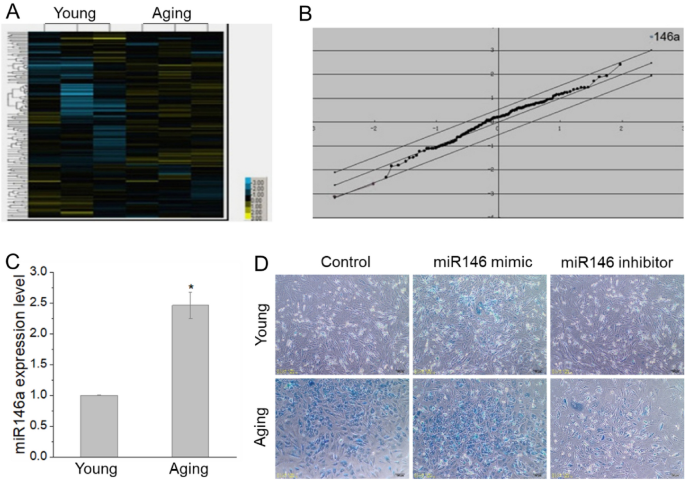
miRNA146a is involved in the aging of BMSCs. The expression profile of miRNAs and the screened results showed that miRNA146a significantly increased in aging BMSCs (A, B), and the results of real time-PCR for miRNA146a confirmed it (C). After transfected with miR146a inhibitor, senescent cells (blue) become less than the group transfected with control oligonucleotide, while senescent cells (blue) increased with miR146a mimic transfecting (D). *P < 0.05. Young young cells, Aging aged cells
Specific miRNA inhibitor and mimic were used to examine the role of miR-146a. The observed increase in SA-β-gal activity was significantly reduced in anti-miR-146a-transfected BMSCs, while increased in miR146a mimic transfected BMSCs, which were compared with control oligonucleotide-transfected BMSCs (Fig. 2D).
To test the effects of miR-146a on the proliferation and differentiation of BMSCs, the results of cck-8 showed that growth ability of BMSCs increased after transfected with miR146 inhibitor (Fig. 3A). Three lineages differentiation was induced with or without miR-146a inhibitor transfection. Inhibition of miR-146a significantly increased all three lineages differentiation (Fig. 3B). Furthermore, over-expression of miR-146a decreased mRNA levels for differentiated marker genes (OPN, OGC, Col1, RUNX-2, PPAR-γ, adiponectin, Col2a1 and Sox9) in BMSCs from aging model, whereas miR-146a inhibitor increased the expression of these marker genes (Fig. 3C). These data indicated that depletion of miR-146a reverse senescence of BMSCs.
Fig. 3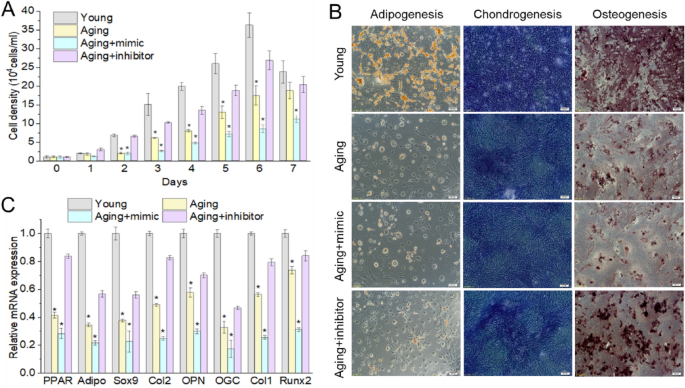
miR146a caused the stemness loss of BMSCs. After transfection of miR146a inhibitor in aging BMSCs, the proliferation (A) and differentiation (B, C) ability of BMSCs increased significantly. *P < 0.05, **P < 0.01, compare with Young group. Young young cells, Aging aged cells, Aging + mimic aged BMSCs transfected with miR146a mimic, Aging + inhibitor aged BMSCs transfected with miR146a inhibitor
miR-146a regulates BMSC aging through CASK
We then predicted the target genes of miR-146a using miRanda database, Pictar and Targetscan. To eliminate false positive rates of the target prediction databases, only the miRNA–mRNA pairs simultaneously predicted at least by two of them were considered as target relationship miRNA–mRNA pairs in our study. Next, we screened the coincidence gene of mRNA expression profiling and prediction databases. mRNA expression profiling showed 165 genes were significantly altered in the BMSCs from D-gal-induced aging rats (P < 0.05, fold change ± 1.2). Among these, 105 genes were significantly up-regulated and 60 genes were markedly down-regulated. Since miRNAs act as negative regulators, up-regulated miRNAs resulted in down-regulated target mRNAs. Therefore, we focused on down-regulated mRNAs, Lipa and CASK were two higher down-regulated gene, and may be a satisfied target gene of miR-146a, from the results of RT-PCR, it indicated that only CASK was down-regulated in BMSC from D-gal-induced aging rats (Fig. 4A).
Fig. 4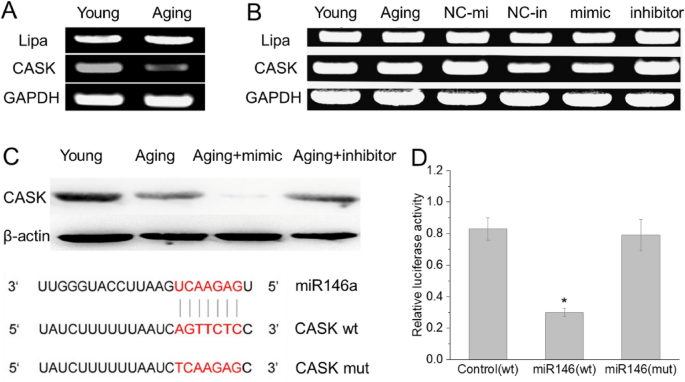
CASK is one of miRNA146a target. PCR results validated the decreased expression of CASK in aging BMSCs (A); The expression of cask in BMSCs showed opposite changes with miR146a after transfection with miR146a mimic and inhibitor (B); Luciferase assay (D) validated that miR-146a inhibit CASK translation by interacting directly with the CASK-3′UTR (C)
To determine whether CASK is a targeted gene of miR-146a, transfection assay with miR-146a mimic and inhibitor was carried out using BMSCs from aging rats. Western-blotting result revealed that the CASK protein expression was significantly decreased with the miR-146a mimic transfection, while the CASK expression levels were significantly increased by miR-146a inhibitor (Fig. 4B). These data indicated that the CASK protein expression levels are controlled by miR-146a, which suggesting that CASK may be the target of miR-146a.
miRNAs inhibit mRNA expression by binding the 3′-UTR of target mRNA. The mRNA of CASK contains one putative miR-146a binding site at its 3’-UTR. (Fig. 4C). To determine whether CASK is the target of miR-146a, we applied the luciferase report gene assay using the pMIR-REPORT Luciferase reporter. Results showed a dramatically lower expression of luciferase in the presence of miR-146a than in the presence of a negative control and a blank control (Fig. 4C). After the mutation of the miR-146a-binding site in the CASK-3′UTR using site-directed mutagenesis, the inhibitory effect of miR-146a was completely eliminated (Fig. 4C). These results demonstrated that miR-146a can inhibit CASK translation by interacting with the CASK-3′UTR.
miR-146a regulates BMSC aging through CASK
We next performed the overexpression and RNAi knock down of CASK to identify the role of CASK on cell proliferation and differentiation. Expression of endogenous CASK protein was efficiently inhibited after transfection with siRNA expressed constructs, the depletion resulting in a significant increase of senescent cells and dead cells, while overexpression of CASK resulted in decrease of senescent cells (Fig. 5A), while increased the cell growth (Fig. 5B) and differentiation ability (Fig. 5C, D). Together, these results indicate that CASK may play an important role in stimulating cell growth and differentiation.
Fig. 5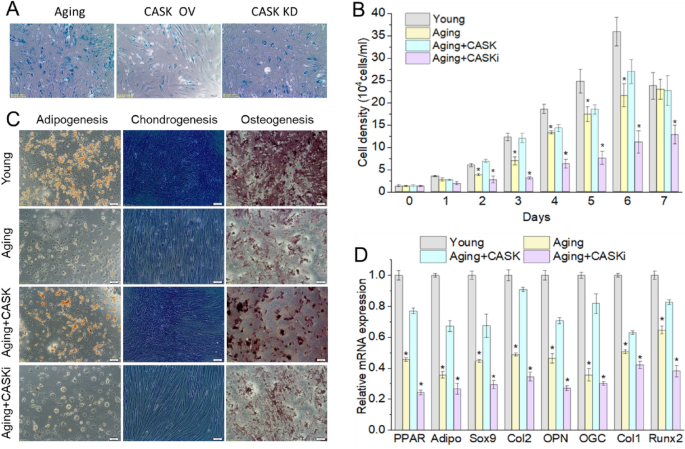
Cask can reverse the stemness of BMSCs. After knock down cask with siRNA, aging BMSCs showed more senescent cells and dead cells, while overexpressing cask in aging BMSCs, senescent cells decreased significantly (A). Proliferation (B) and differentiation (C, D) ability of BMSCs were decreased in D-galactose induced aging group compare with control, after transfection of cask in aging BMSCs, the proliferation and differentiation ability increased again. Aging aging BMSCs transfected empty vector as control, CASK KD knock down cask with siRNA in aging BMSCs, CASK OV overexpression cask in aging BMSCs. *P < 0.05, **P < 0.01, compare with Young group. Young young cells, Aging aged cells, Aging + CASK aged BMSCs transfected with plasmid CASK, Aging + CASKi aged BMSCs transfected with CASK siRNA
Discussion
Aging has been associated with a decreased stem cell function (De et al., 2021), studies already indicated that the function of adult stem cell declines with age in all tissue types. The dysfunction of aged stem cells may result from accumulation of irreversible modifications, including genetic alterations, mitochondrial lesions, and telomere shortening (Rudolph et al., 2021). Importantly, new evidence supports the notion that adult stem cell senescence and aging are also regulated by specific epigenetic modifications.
In order to investigate the epigenetic modification of BMSC during aging, we treated rats daily with D-galactose to induce aging and investigate BMSC senescence in vivo, because D-galactose induced mouse and rat aging model has been widely used for studying aging mechanisms and screening drugs (Liu et al., 2021; Ye et al., 2022). After we induced rat aging with D-galactose, the age-associated oxidative biomarkers changed significantly. The increased level of MDA and decreased T-SOD and GSH-Px activities indicated that the aging happened in rats. Compared with BMSCs from normal rats, those from aging rats have more senescent cells with a decline in their clonogenic and proliferation capacity. For aging BMSCs, the numbers do not necessarily decline with age, but the cellular function is clearly affected (Bustos et al., 2014; Guo et al., 2021). Our aging model also showed that BMSCs from aging rat have less differentiation ability than BMSCs from normal rats. All these results demonstrated that D-galactose induced aging is an ideal model to study the molecular mechanisms of BMSC aging and age-associated degenerative disease.
Cellular aging has been attributed to various mechanisms, recent studies suggest that miRNAs are important regulators of senescence. miR-34a and miR-93 were found to be overexpressed in the rat aging liver (Li et al., 2011), and miR-34a was shown to induce endothelial progenitor cell senescence (Zhao et al., 2010). Furthermore, miR-449 was found to induce senescence and apoptosis, as well as to inhibit cell proliferation by activation of p53 pathway (Bou Kheir et al., 2011). Studies also showed that miRNAs may participate in controlling stem cells replicative senescence. Changes in the miRNA expression profile were found in replicative senescent human MSCs (Bi et al., 2022). An aging-related miRNA, miR-29b-5p was found to recruit synovial stem cell and suppress its senescence for successful cartilage repair (Zhu et al., 2022). DNA methyltransferase controls stem cell aging by regulating BMI1 and EZH2 through microRNAs (So et al., 2011). All of these indicate that stem cell aging and senescence are related with miRNA. However, specific miRNAs and their target genes in the regulation of BMSC senescence in vivo have not been well characterized and poorly understood. Therefore, we profiled microRNA in aging BMSCs, and found that miR-146a was upregulated significantly, in order to confirm the role of miR-146a on BMSCs aging, up and down regulated miR-146a in aging BMSCs were performed, the results demonstrated that miR-146a play an important role on BMSC senescence, and regulate the proliferation and differentiation of BMSCs. miR-146a is primarily involved in the regulation of inflammation and function in the innate immune system (Mortazavi-Jahromi., 2020). miR-146 has also been reported to be highly upregulated in osteoarthritis cartilage (Papathanasiou., 2019; Zhang., 2021), and could be involved in its pathogenesis. Recently, Fabiola reported that miR-146a is marker of senescence-associated pro-inflammatory status in cells involved in vascular remodeling. They found that an up-regulation of miR-146a was expressed not only in HUVECs, but also in HAEC and in HCAEC senescent cells (Fabiola et al., 2013). Our study also indicated that up-regulation miR-146a caused the senescence of BMSC and the loss of its stemness.
In order to know how miR-146a regulate the aging of BMSC and what are the target genes of miR-146a, we predicted the target genes of miR-146a using miRanda database, and screened the coincidence gene of mRNA expression profiling and prediction databases, finally, Calcium/calmodulin-dependent serine protein kinase (CASK) was found a possible target gene of miR-146a. CASK mRNA and protein expression were significantly down-regulated with the miR-146a mimics transfection, and up-regulated by miR-146a inhibitor, which indicated that CASK may be controlled by miR-146a. Because miRNA negatively regulate gene expression by binding to the 3′-untranslated region of their target genes for cleavage or translational repression (Bartel et al., 2009), luciferase reporter assays showed miR-146a is able to inhibit cask translation by interacting directly with the CASK-3’UTR, which confirmed that cask is the target gene of miR-146a.
CASK belongs to the membrane-associated guanylate kinase protein family, and function as multiple domain scaffolding protein. Based on its multidomain structure, the ubiquitously expressed CASK participates in multiple interactions, such as protein organization, synaptic transmission, cell junction assembly, transcription regulation and in many extracellular and intracellular signaling pathways (Yang et al., 2022). Besides, CASK interacts with inhibitor of differentiation 1(Id1) through the GUK domain to regulate cell proliferation (Qi et al., 2005), and CASK regulation of cell growth might involve p21 expression (Sun et al., 2009). miR-1587 binding with CASK may be involved in intracellular metabolic and transcriptional physiological processes (Zhang et al., 2021a, 2021b). In order to study the effect of CASK on BMSC aging and stemness, overexpression of CASK was performed, CASK not only can rescue the senescent cells, the proliferation and differentiation abilities of BMSCs also be reversed by CASK. All these results demonstrated that miR-146a directly interacts with CASK to regulate the aging of BMSCs.
In conclusion, we demonstrated for the first time that miR-146a plays a critical role in regulating BMSC senescence by targeting CASK expression. The regulation of CASK by miR-146a enables us to explain how BMSCs become senescence after D-gal-induction, and also suggest another linking mechanism between miR-146a and BMSCs senescence. Extending this study, it would be meaningful to find the connection between CASK and cellular senescence, and confirm the function of CASK in cellular senescence.
Availability of data and materials
Not applicable.
Code availability
Not applicable.
References
Bartel, D. P. (2009). MicroRNAs: Target recognition and regulatory functions. Cell, 136(2), 215–233.
Bi, Y., Qiao, X., Liu, Q., Song, S., Zhu, K., Qiu, X., Zhang, X., Jia, C., Wang, H., Yang, Z., Zhang, Y., & Ji, G. (2022). Systemic proteomics and miRNA profile analysis of exosomes derived from human pluripotent stem cells. Stem Cell Research & Therapy, 13(1), 449.
Boehm, M., & Slack, F. (2005). A developmental timing microRNA and its target regulate life span in C. elegans. Science, 310(5756), 1954–1957.
Bonifacio, L. N., & Jarstfer, M. B. (2010). MiRNA profile associated with replicative senescence, extended cell culture, and ectopic telomerase expression in human foreskin fibroblasts. PLoS ONE, 5(9), 12489–12489.
Bou Kheir, T., Futoma-Kazmierczak, E., Jacobsen, A., Krogh, A., Bardram, L., Hother, C., Grønbæk, K., Federspiel, B., Lund, A. H., & Friis-Hansen, L. (2011). miR-449 inhibits cell proliferation and is down-regulated in gastric cancer. Molecular Cancer, 10, 29.
Bustos, M. L., Luai, H., Kapetanaki, M. G., Lino-Cardenas, C. L., & Lyle, M. (2014). Aging mesenchymal stem cells fail to protect because of impaired migration and anti-inflammatory response. American Journal of Respiratory and Critical Care Medicine, 189(7), 787–798.
Cai, Y., Song, W., Li, J., Jing, Y., Liang, C., Zhang, L., Zhang, X., Zhang, W., Liu, B., An, Y., Li, J., Tang, B., Pei, S., Wu, X., Liu, Y., Zhuang, C. L., Ying, Y., Dou, X., Chen, Y., … Liu, G. H. (2022). The landscape of aging. Science China Life Sciences, 65(12), 2354–2454.
Cavalli, G., & Heard, E. (2019). Advances in epigenetics link genetics to the environment and disease. Nature, 571(7766), 489–499.
De, D., Karmakar, P., & Bhattacharya, D. (2021). Stem cell aging and regenerative medicine. Advances in Experimental Medicine and Biology, 1326, 11–37.
Demirel-Yalciner, T., Sozen, E., & Ozer, N. K. (2022). Endoplasmic reticulum stress and miRNA impairment in aging and age-related diseases. Front Aging, 2, 790702.
Duan, R., Fu, Q., Sun, Y., & Li, Q. (2022). Epigenetic clock: A promising biomarker and practical tool in aging. Ageing Research Reviews, 81, 101743.
Fabiola, O., Raffaella, L., Rina, R., Fiorella, M., & Maria, R. R. (2013). MiR-146a as marker of senescence-associated pro-inflammatory status in cells involved in vascular remodeling. Age, 35(4), 1157–1172.
Ghafouri-Fard, S., Abak, A., Talebi, S. F., Shoorei, H., Branicki, W., Taheri, M., & Akbari, D. N. (2021). Role of miRNA and lncRNAs in organ fibrosis and aging. Biomedicine & Pharmacotherapy, 143, 112132.
Guo, Y., Jia, X., Cui, Y., Song, Y., Wang, S., Geng, Y., Li, R., Gao, W., & Fu, D. (2021). Sirt3-mediated mitophagy regulates AGEs-induced BMSCs senescence and senile osteoporosis. Redox Biology, 41, 101915.
Kasper, G., Mao, L., Geissler, S., Draycheva, A., Trippens, J., Kühnisch, J., Tschirschmann, M., Kaspar, K., Perka, C., Duda, G. N., & Klose, J. (2009). Insights into mesenchymal stem cell aging: Involvement of antioxidant defense and actin cytoskeleton. Stem Cells, 27(6), 1288–1297.
Li, N., Muthusamy, S., Liang, R., Sarojini, H., & Wang, E. (2011). Increased expression of miR-34a and miR-93 in rat liver during aging, and their impact on the expression of Mgst1 and Sirt1. Mechanisms of Ageing and Development, 132(3), 75–85.
Liu, J., Gao, J., Liang, Z., Gao, C., Niu, Q., Wu, F., & Zhang, L. (2022). Mesenchymal stem cells and their microenvironment. Stem Cell Research & Therapy, 13(1), 429.
Liu, Y., Liu, Y., Guo, Y., Xu, L., & Wang, H. (2021). Phlorizin exerts potent effects against aging induced by D-galactose in mice and PC12 cells. Food & Function, 12(5), 2148–2160.
López-Otín, C., Blasco, M. A., Partridge, L., Serrano, M., & Kroemer, G. (2023). Hallmarks of aging: An expanding universe. Cell, 186(2), 243–278.
Mortazavi-Jahromi, S. S., Aslani, M., & Mirshafiey, A. (2020). A comprehensive review on miR-146a molecular mechanisms in a wide spectrum of immune and non-immune inflammatory diseases. Immunology Letters, 227, 8–27.
Papathanasiou, I., Trachana, V., Mourmoura, E., & Tsezou, A. (2019). DNA methylation regulates miR-140-5p and miR-146a expression in osteoarthritis. Life Sciences, 228, 274–284.
Qi, J., Su, Y. Y., Sun, R. J., Zhang, F., Luo, X. F., Yang, Z. C., & Luo, X. D. (2005). CASK inhibits ECV304 cell growth and interacts with Id1. Biochemical and Biophysical Research Communications, 328(2), 517–521.
Rudolph, K. L. (2021). Stem cell aging. Mechanisms of Ageing and Development, 193, 111394.
So, A. Y., Jung, J. W., Lee, S., Kim, H. S., & Kang, K. S. (2011). DNA methyltransferase controls stem cell aging by regulating BMI1 and EZH2 through microRNAs. PLoS ONE, 6(5), e19503.
Sun, R., Su, Y., Zhao, X., Qi, J., Luo, X., Yang, Z., Yao, Y., Luo, X., & Xia, Z. (2009). Human calcium/calmodulin-dependent serine protein kinase regulates the expression of p21 via the E2A transcription factor. The Biochemical Journal, 419(2), 457–466.
Yamakawa, H., Kusumoto, D., Hashimoto, H., & Yuasa, S. (2020). Stem cell aging in skeletal muscle regeneration and disease. International Journal of Molecular Sciences, 21(5), 1830.
Yang, K., Lin, L., Yuan, F., Li, X., Liu, Z., Lan, X., Wang, Y., Ren, Y., Li, J., & Chen, Y. (2022). Two heterozygous mutations in the calcium/calmodulin-dependent serine protein kinase gene (CASK) in cases with developmental disorders. Molecular Genetics & Genomic Medicine, 10(11), e2065.
Ye, M., Liu, J., Deng, G., Cai, X., Zhang, X., Yao, L., Wu, J., He, X., Peng, D., & Yu, N. (2022). Protective effects of Dendrobium huoshanense polysaccharide on D-gal induced PC12 cells and aging mice, in vitro and in vivo studies. Journal of Food Biochemistry, 46(12), e14496.
Zhang, H., Shang, H., Wang, Z., & Li, K. (2023). Associations of miRNA-146a and miRNA-223 with rheumatoid arthritis and their predictive values. Int J Gen Med, 16, 3211–3218.
Zhang, H., Zheng, W., Li, D., & Zheng, J. (2021a). miR-146a-5p promotes chondrocyte apoptosis and inhibits autophagy of osteoarthritis by targeting NUMB. Cartilage, 13(2), 1467S-1477S.
Zhang, L., Lu, Q., & Chang, C. (2020). Epigenetics in health and disease. Advances in Experimental Medicine and Biology, 1253, 3–55.
Zhang, L., Zhou, J., Xu, M., & Yuan, G. (2021b). Exploration of the Hsa-miR-1587-protein interaction and the inhibition to CASK. International Journal of Molecular Sciences, 22(19), 10716.
Zhao, T., Li, J., & Chen, A. F. (2010). MicroRNA-34a induces endothelial progenitor cell senescence and impedes its angiogenesis via suppressing silent information regulator 1. American Journal of Physiology, Endocrinology and Metabolism, 299(1), 110–116.
Zhu, J., Yang, S., Qi, Y., Gong, Z., Zhang, H., Liang, K., Shen, P., Huang, Y. Y., Zhang, Z., Ye, W., Yue, L., Fan, S., Shen, S., Mikos, A. G., Wang, X., & Fang, X. (2022). Stem cell-homing hydrogel-based miR-29b-5p delivery promotes cartilage regeneration by suppressing senescence in an osteoarthritis rat model. Science Advances, 8(13), eabk0011.
Zhu, Y., Liu, T., Song, K., Fan, X., Ma, X., & Cui, Z. (2008). Adipose-derived stem cell: A better stem cell than BMSC. Cell Biochemistry and Function, 26(6), 664–675.
Funding
This study was supported by National Natural Science Foundation of China (82272292); Natural Science Foundation of Guangdong Province (2021A1515011155); Shenzhen Basic Research Program (JCYJ20220531101805012).
Author information
Authors and Affiliations
Shenzhen Key Laboratory for Anti-Aging and Regenerative Medicine, School of Basic Medical Sciences, Shenzhen University Medical School, Shenzhen University, Shenzhen, 518055, Guangdong, China
Yanxia Zhu, Jia Liu, Yiyang Liu, Junyu Su, Kangling Xu & Guangqian Zhou
Contributions
YZ and GZ designed the study; YZ, HL and JL performed the experimental work; YL and JS carried out the animal experiments; YZ and GZ wrote the paper; YZ, YL, JS, KX, and HL assisted in the experimental process and contributed analytic tools. All authors read and approved the final manuscript.
Corresponding authors
Correspondence to Yanxia Zhu or Guangqian Zhou.
Ethics declarations
Conflict of interest
The authors declare no potential conflicts of interest.
Ethics approval
Not applicable.
Consent to participate
Not applicable.
Consent for publication
Not applicable.
Rights and permissions
Springer Nature or its licensor (e.g. a society or other partner) holds exclusive rights to this article under a publishing agreement with the author(s) or other rightsholder(s); author self-archiving of the accepted manuscript version of this article is solely governed by the terms of such publishing agreement and applicable law.
About this article
Cite this article
Zhu, Y., Liu, J., Liu, Y. et al. MiR-146a induces the aging of mesenchymal stem cell via CASK. GENOME INSTAB. DIS. 5, 35–44 (2024). https://doi.org/10.1007/s42764-023-00119-z
Received10 October 2023
Revised27 November 2023
Accepted06 December 2023
Published17 January 2024
Issue DateFebruary 2024
DOIhttps://doi.org/10.1007/s42764-023-00119-z
Share this article
Anyone you share the following link with will be able to read this content:
Get shareable link
用户登录
还没有账号?
立即注册